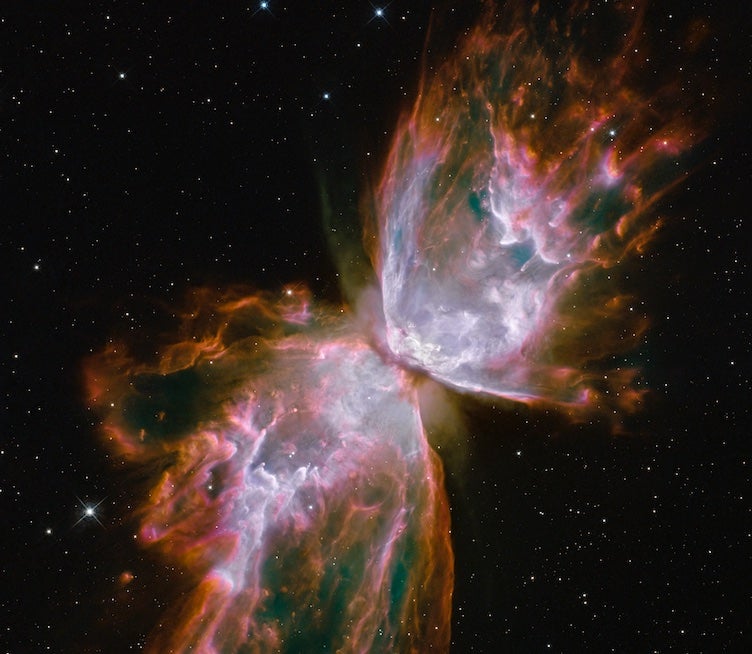
Whether you realize it or not, you’re part of a revolution.
Let me explain. In the past, ideas to interpret the nature of things developed slowly. Before there was astronomy, there was astrology, attempting to divine mystic meaning from the motions of stars and planets. What became chemistry was originally alchemy, seeking to transform lead into gold.
It took millennia for these primitive practices to mature into the sciences. And even when they did, early science was largely about classification, whether it be bones, mineral specimens, or tree leaves.
The landscape of astronomy really changed in the 1860s, when the development of spectroscopy ushered in a new era of understanding the universe: the era of astrophysics. Finally, astronomers could begin in earnest to decipher the physical nature of objects in the cosmos in an objective and measured sense.
Big moments of realization followed. A century ago, Albert Einstein transformed physics with his theories of relativity, rewriting our basic laws of motion for the first time since Isaac Newton. Edwin Hubble revolutionized astronomy in the early 1920s by discovering the nature of galaxies and the first glimmers of the enormous cosmic distance scale.
But the most recent generation has witnessed an explosion of knowledge about the universe like nothing that preceded it. And along the way, huge surprises have completely changed many of our ideas about the universe.
We are now getting answers to some of the largest and most fundamental questions that humans have asked since time immemorial: How old is the universe? How did it begin? How large is the cosmos? Do black holes exist? How will the universe end? And why are we here on a planet orbiting a pretty ordinary star?
Truly, there has never been a better time to be someone who loves astronomy and gazing up at the heavens in awe.
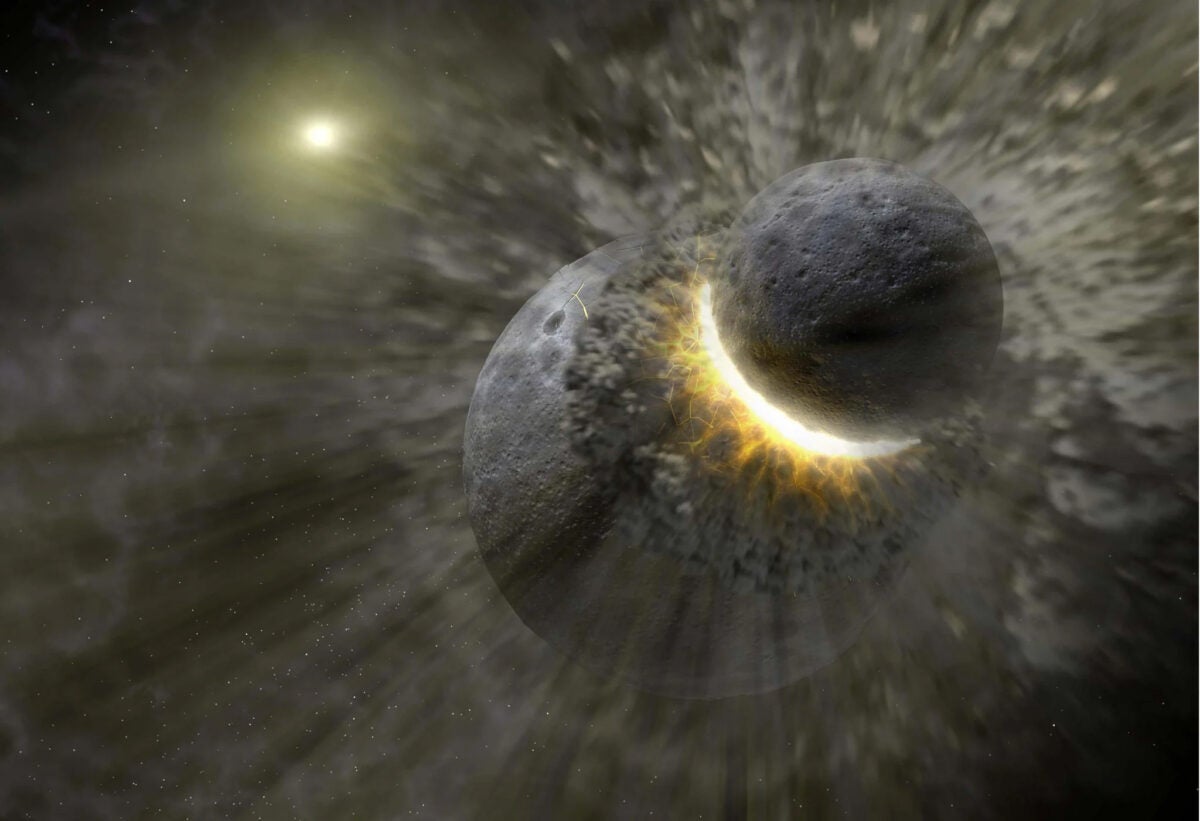
1. How the Moon formed
One of the great mysteries of astronomy is our nearest celestial neighbor. Presumably among the first objects in the sky that our ancient ancestors noticed and wondered over is the Moon.
For a long time, the Moon presented planetary scientists with some perplexing puzzles. The Earth-Moon system is a strange one. It has high angular momentum — that is, it has a high orbital speed, rotational speed, and mass. The Moon is also relatively massive compared to Earth and its orbit is odd, inclined by 5.1° to the ecliptic plane. Yet, for all that mass, the Moon has a strangely low overall density.
The first strong hints about the Moon’s origin arrived when the Apollo astronauts brought back Moon rocks to study — though at first, the samples only added to the mysteries. The rocks’ chemical complexity confused scientists and led to several possibilities for the Moon’s origin. Perhaps the Moon was a roaming body captured by Earth’s gravity. Or maybe Earth and the Moon formed simultaneously as a sort of double planet. Perhaps our world gave birth to the Moon through an act of fission. Maybe large planetesimals in Earth’s neighborhood, early in the solar system’s history, broke apart and formed the Moon. Or maybe a large impact occurred, generating debris that formed the Moon we now have.
In the 1970s, William Hartmann and Donald Davis at the University of Arizona suggested that a Mars-sized body (later called Theia) collided with Earth in the solar system’s early days, knocking out a huge cloud of material that accreted into the Moon. At first, the idea failed to catch on. But after years of study, the idea took hold, with further strong evidence provided by analysis in the 1990s by planetary scientist Robin Canup.
The so-called Giant Impact Hypothesis is now the leading idea about how the Moon formed. The strongest line of evidence is that oxygen isotopes — the particular varieties of the element, distinguished by their number of neutrons — in Moon rocks closely match those of Earth. They must have come from a common source, planetary scientists believe, and an impact would explain that.
What happened to Theia? Most of it was absorbed into Earth, under your feet.
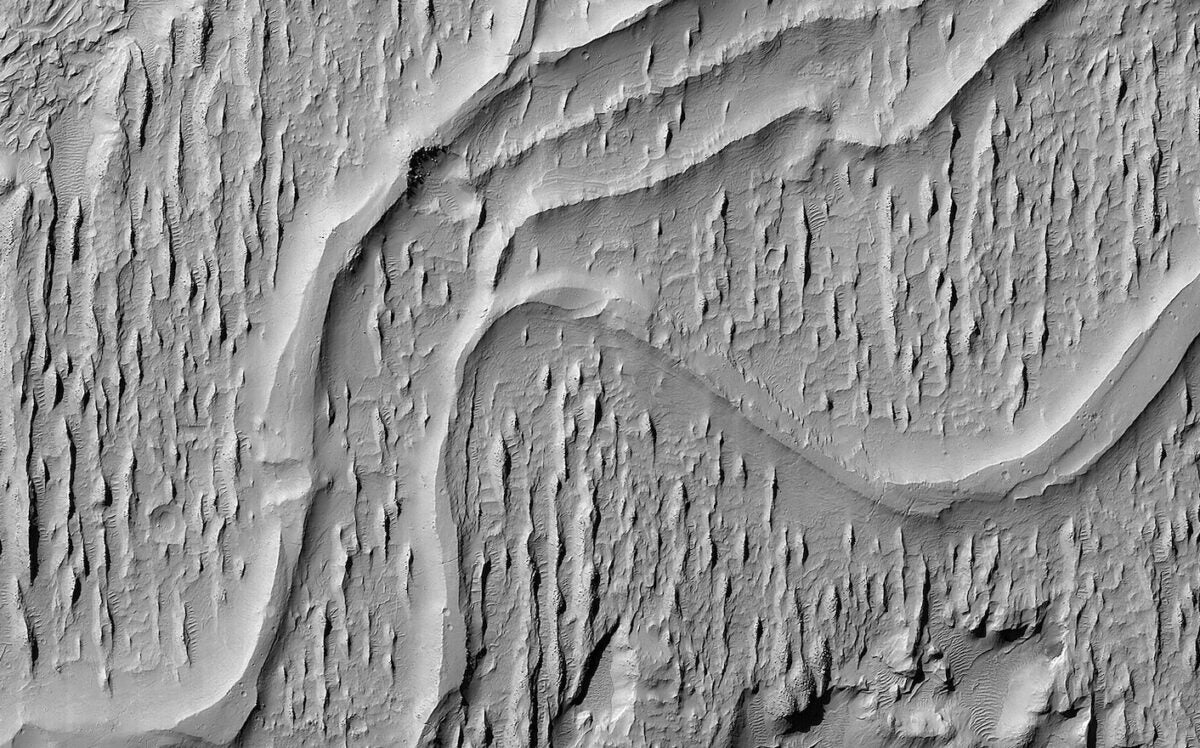
2. Where did the water on Mars go?
Science fiction dating back to the 19th century held that Mars was an Earth-like world, perhaps with all manner of creatures staring back at us. Our planetary neighbor had a complex system of canals — or so believed Percival Lowell, armed with his mighty 24-inch telescope in Arizona. The lore built around the Red Planet had us hoping that this world might hold forests, water-worn valleys, and cousins to humankind among its living beings.
Any possibility of that was shattered when the first spacecraft imaged Mars up close in the 1960s. It was obviously a barren, lifeless world.
But with the arrival of orbiters and rovers at the Red Planet in the 1990s, a deeper story of Mars’ evolution began to emerge. These craft, particularly the Mars Reconnaissance Orbiter, demonstrated that abundant water once had flowed on the martian surface. Substantial river channels are carved into the rocks in many places. There’s plentiful evidence for subsurface aquifers on Mars, as well as water ice, including in the polar caps. But none of that water is flowing on the planet’s surface. So what caused the planet to dry up?
The short answer is climate change. Planetary scientists believe that during its early history, Mars had a much denser atmosphere than it does now, which helped to keep water on the planet’s surface. But then the atmosphere evolved and thinned. Now, water molecules on the surface of Mars would evaporate and quickly be broken apart into lighter byproducts, which are then quickly lost into space.
It will be intriguing for future astronauts on Mars to drill down into the aquifers just yards below the surface. Could they contain microbes? It might herald the first detection of life on another planet.
3. Why did Venus turn inside out?
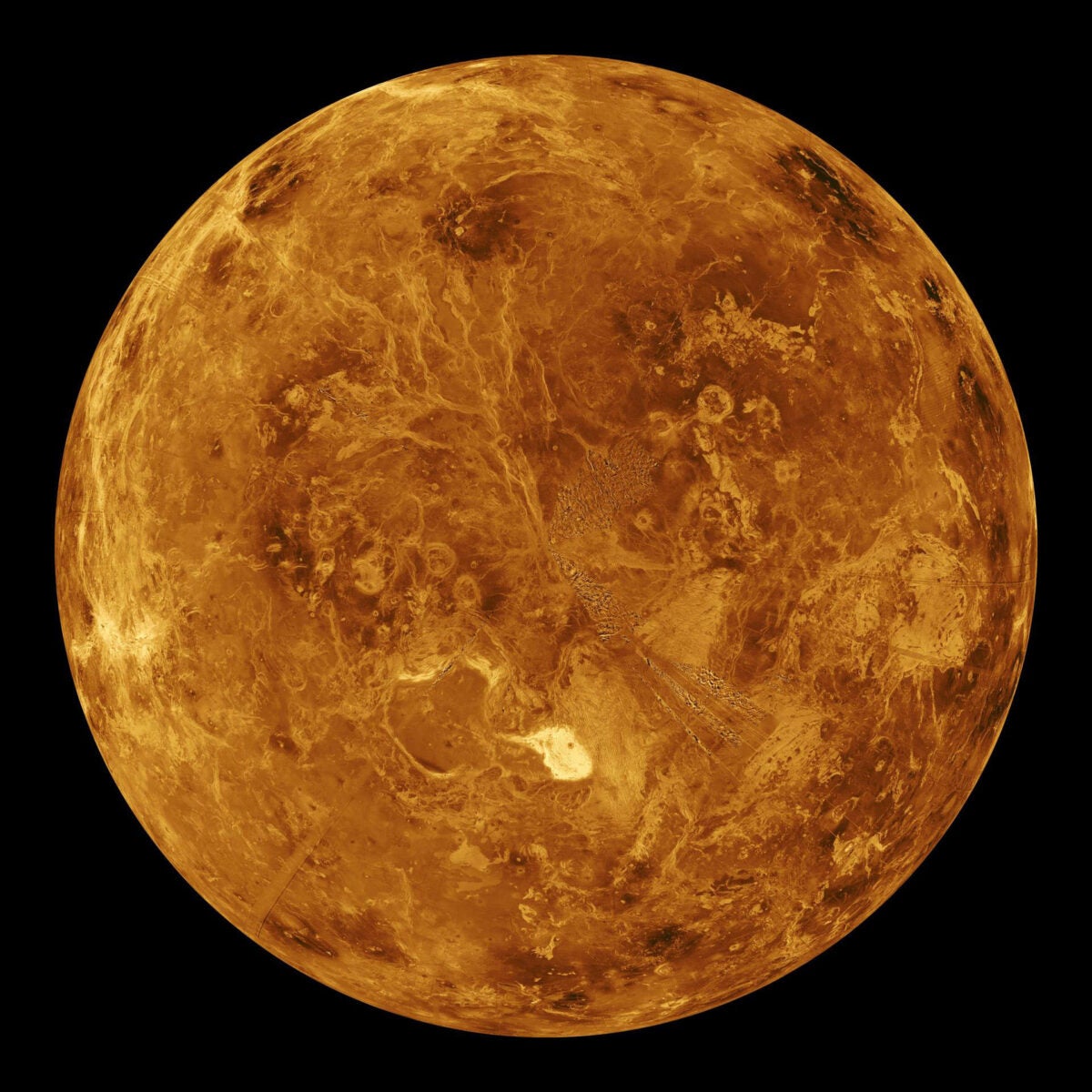
The brightest object in our skies after the Sun and Moon, Venus is another planetary neighbor filled with rich lore and science fiction fun. As with Mars, we discovered with the first spacecraft forays that Venus is not exactly a place to hang around. Soviet and American landers in the 1960s quickly found that they could survive on the planet’s surface for only a short time, subjected to temperatures of 800 degrees Fahrenheit (425 degrees Celsius). That Venus is like the cultural fables of hell became immediately apparent, a resemblance that only deepened with the knowledge of its toxic atmospheric gases.
Besides disappointing legions of science fiction fans, Venus also posed a substantial puzzle for planetary scientists. Researchers typically estimate the ages of planetary surfaces by counting craters. We know that the inner solar system underwent a violent epoch called the Late Heavy Bombardment, in which small bodies pelted the surfaces of planets and moons. Evidence of this still exists in a simple glance at the Moon. But Venus, by comparison, shows very few impact craters. “What is going on with Venus?” planetary scientists wondered.
A great deal of data on Venus and its surface arrived in the early 1990s with the Magellan spacecraft, kicking off a new era of Venus studies. It became apparent that Venus is a planet with a young surface. It has been very volcanically active in the relatively recent past. And perhaps three-quarters of a billion years ago, Venus was globally resurfaced via a cataclysmic volcanic event. The older craters were obliterated. As one planetary scientist put it, “Venus is the planet that threw up on itself.”
What could have caused this dramatic action? The belief is that an enormous amount of energy was trapped deep within Venus, perhaps because its crust grew so thick it smothered the world in its own internal heat. At some critical point, this energy escaped and, as Venus has no plate tectonics, the event led to the cataclysmic global resurfacing.
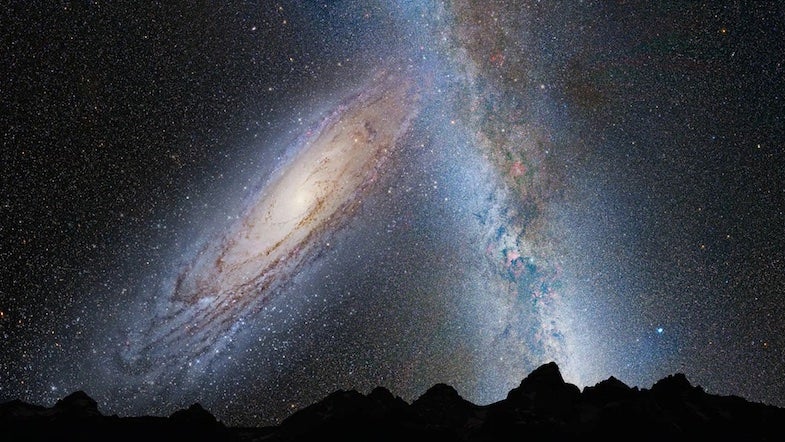
4. Here comes Milkomeda
Mysteries and strange discoveries also extend far out into the cosmos beyond our solar system. In the early 1920s, Edwin Hubble discovered the nature of galaxies when he found a Cepheid variable star in the Andromeda Galaxy, our closest big extragalactic neighbor. Because Cepheid variables pulsate at a rate that depends on their intrinsic brightness, Hubble could accurately determine its distance.
He found that the Andromeda Galaxy lies an astonishing 2.5 million light-years away. The light you see from the galaxy in your telescopic eyepiece has been traveling at the fastest possible speed for 2.5 million years, since the time our early ancestors roamed Earth.
Even before Hubble, the astronomer V.M. Slipher at Lowell Observatory had found that most “spiral nebulae,” as they were then called, are moving away from each other.
But not all of them. The exceptions are usually within clusters and groups. We are in the same group of galaxies with Andromeda, named the Local Group by Hubble. Gravity and random motions play a large role in the behavior of galaxies in groups.
It turns out that the Andromeda Galaxy and our own Milky Way are moving toward each other at a velocity of about 70 miles per second (110 km/s). In 2008, Harvard University astronomy professor Avi Loeb and his collaborators produced a detailed study of the Andromeda Galaxy and found that eventually the Andromeda Galaxy and the Milky Way will merge into one supergalaxy, which they dubbed Milkomeda.
There’s nothing to worry about — this will happen long after life on Earth is gone. But eventually, several billion years from now, the Andromeda Galaxy will loom larger and larger in our sky — or in the skies of surviving planets in the Milky Way. Any inhabitants will witness the galaxies’ spiral arms entangle in a cosmic dance, and perhaps Milkomeda will eventually appear like one of the great elliptical galaxies we can now observe, such as Centaurus A in our southern sky.
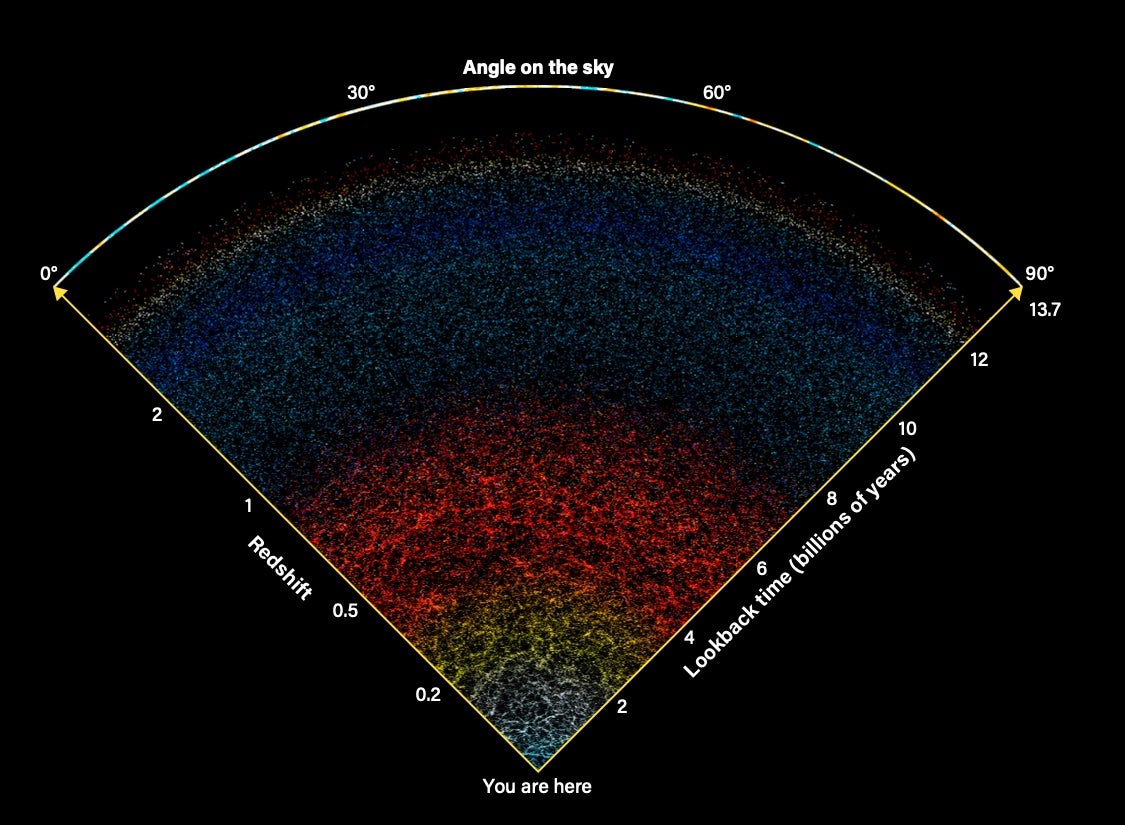
5. How large is the universe?
This is a question that in some form must go back to when human ancestors first became capable of complex thought, staring up into the sky and contemplating why they exist. And yet the answer, in any sophisticated sense, has come pretty recently.
Cosmological investigations tell us that the universe is 13.8 billion years old. The most recent and accurate determination of major cosmological data comes from the European Space Agency’s Planck satellite. We’ve known that the Big Bang marks the starting point of the universe since Arno Penzias and Bob Wilson discovered the cosmic microwave background radiation in 1964. We know the universe is expanding, which Slipher discerned in 1912. And since 1998 and the discovery of dark energy (see No. 7), we know that this expansion is accelerating over time.
The amazing fact is that we now also know the cosmos is at least 93 billion light-years in diameter. How can that be? Because the universe is not like a box with its contents moving outward. Space itself expands over time, interstitially. A distance of 1 inch (2.54 centimeters) in the early universe later became 2 inches (5.1 cm), and so on.
Note that I said the universe is at least 93 billion light-years across. That’s because in some cosmological models, the universe could be infinite. Now that sounds like sci-fi — but maybe it’s true.
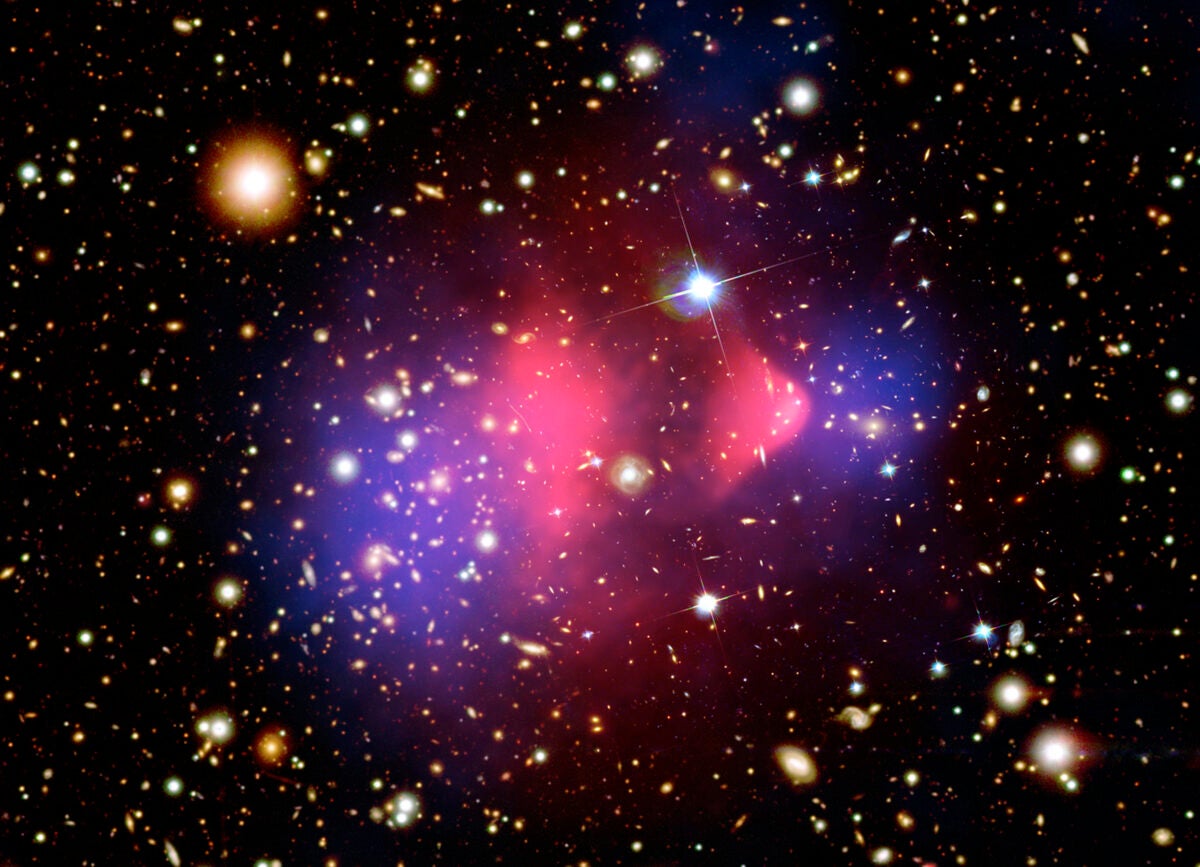
6. The mystery of dark matter
In the early 1930s, two astronomers independently postulated the existence of a strange, unseen form of matter. The Dutch astronomer Jan Oort (soon to be famous for studies of the solar system’s cloud of comets) and Swiss astronomer Fritz Zwicky both studied the motions of stars in our galaxy. They each determined that the bright stuff we can see, the stars and the galactic disk, can’t be everything that exists. Some unseen form of matter must also exist and help the stars to orbit the galaxy’s center as quickly as they do. Zwicky named it dunkle Materie, or dark matter.
Fast-forward 40 years to the 1970s, when American astronomer Vera Rubin and her research group at the Carnegie Institution were busily studying the rotation of galaxies. They found indeed that dark matter exists, and theorists at the time proposed it must exist in the form of unseen particles. In the decades that followed, proposed particles have included WIMPs (weakly interacting massive particles) and axions.
Fast-forward another 40 years to just the last decade or so, and satellites like Planck have determined that this dark matter, whatever it is, must make up some 26 percent of the mass-energy content of the universe. All the familiar stuff in the cosmos — stars, planets, galaxies, dogs, cats, trees, etc. — must be only about 5 percent of the mass-energy. We call that familiar stuff baryonic matter.
That means of all the matter in the universe, about 80 percent consists of dark matter, and we don’t yet understand what it is or what it’s made of. Particle accelerator experiments have not yet detected an axion or any other explanation. Stay tuned.
7. The bigger mystery of dark energy
Wait a minute, you say. What is this mass-energy content you speak of? One of the things Albert Einstein’s theories of relativity taught us, now more than a century ago, is that matter and energy are interconvertible forms of the same thing. That’s what E = mc2 is all about. The c, which represents the speed of light, is a constant. So, the equation essentially shows that energy equals mass. The old joke, only slightly exaggerated (because the conversion doesn’t happen perfectly or instantly), is that you can demonstrate this by eating a sandwich and then running down the street, converting the food into energy.
The Planck satellite and others have helped us to understand the composition of the universe. But our understanding of the universe was again rocked in 1998, when astronomers discovered what is now called dark energy. Through observations of distant supernovae, astronomers found that the expansion of the universe, long believed to be constant, is accelerating over time.
This means that an unknown force — dark energy — is accelerating the expansion. What this force is composed of and its exact nature are still a mystery. But it definitely exists. So, if you are unsettled by the fact that we don’t know what dark matter is, hold onto your hat. Dark energy makes up the remaining 69 percent of the mass-energy content of the cosmos.
A note to up-and-coming cosmologists: If you want to win a Nobel Prize, find out what either dark matter or dark energy is. Your prize will be a sure thing.
8. Black holes are everywhere
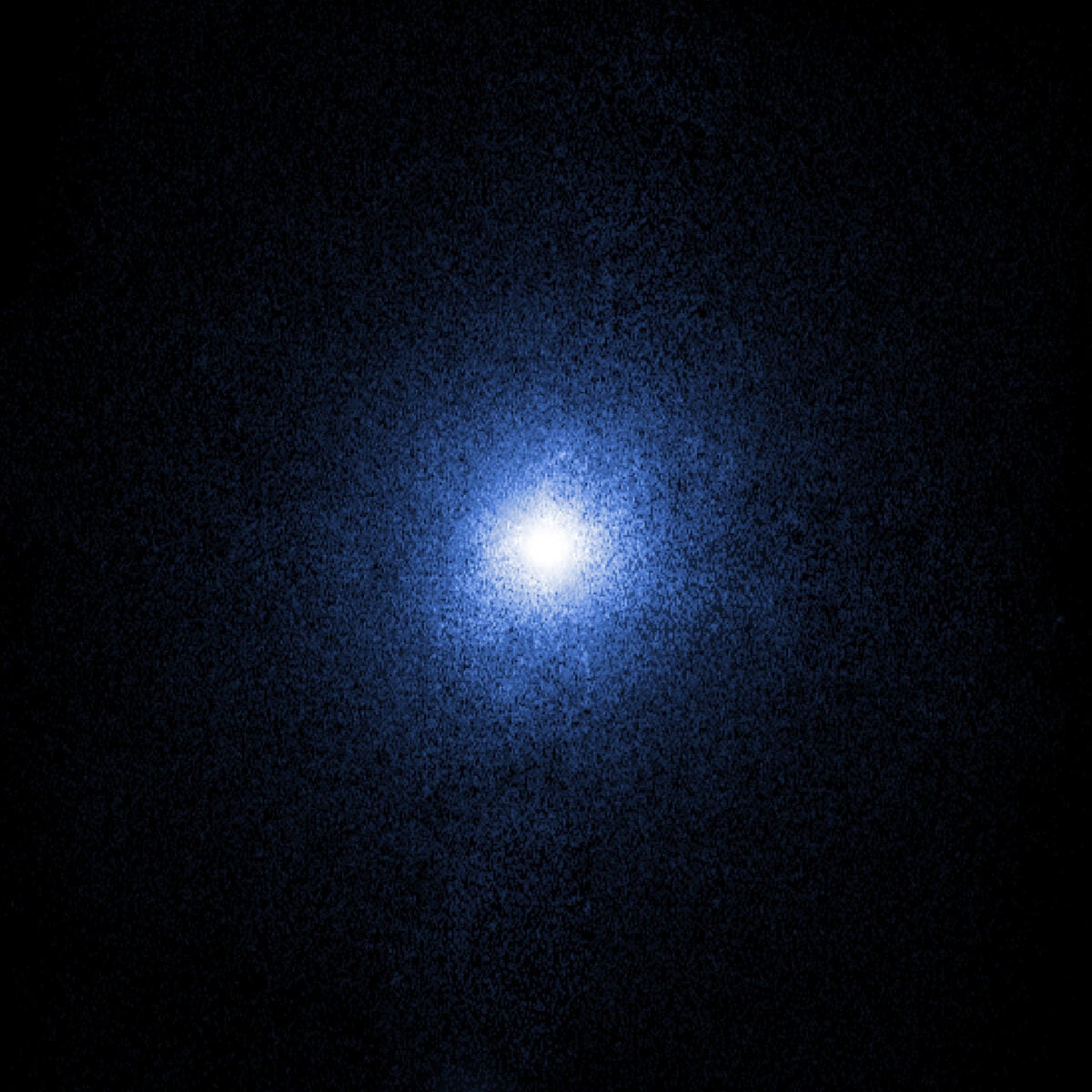
My Starmus festival friend Kip Thorne, the world’s leading expert on black holes, writes that they are “the most luminous objects in the universe, but no light!” These regions of space so gravitationally strong that nothing, not even light, can escape, are among the most bizarre objects in the cosmos. And infalling material gets so hot that it glows in X-rays, which are more energetic than the visible light our eyes are tuned to see.
The concept of black holes goes all the way back to the English philosopher and clergyman John Michell, who wrote about “dark stars” in a paper in 1783. But confirming the existence of black holes was a long time coming. They are extremely hard to find. They are, after all, not luminous.
In the 1970s, astronomers detected an extremely strong X-ray source dubbed Cygnus X-1, seemingly a black hole candidate. Thorne and his friend Stephen Hawking made a famous bet over whether the object would be confirmed as a black hole. Finally, by 1990, it was shown to be a stellar-mass black hole — the remnant of a dead, massive star.
Soon thereafter, astronomers using the Hubble Space Telescope began finding evidence for another type of black hole — supermassive black holes — in the centers of many galaxies. Within the last generation, it’s become clear that massive galaxies have central supermassive black holes. (Smaller dwarf galaxies, however, do not.)
Millions of stellar-mass black holes must exist in the Milky Way, although we know of only a couple of dozen because they remain so hard to detect. The first examples of a third class, intermediate-mass black holes, have also been discovered. The catalog of known black holes will certainly swell into astronomical numbers as research carries on.
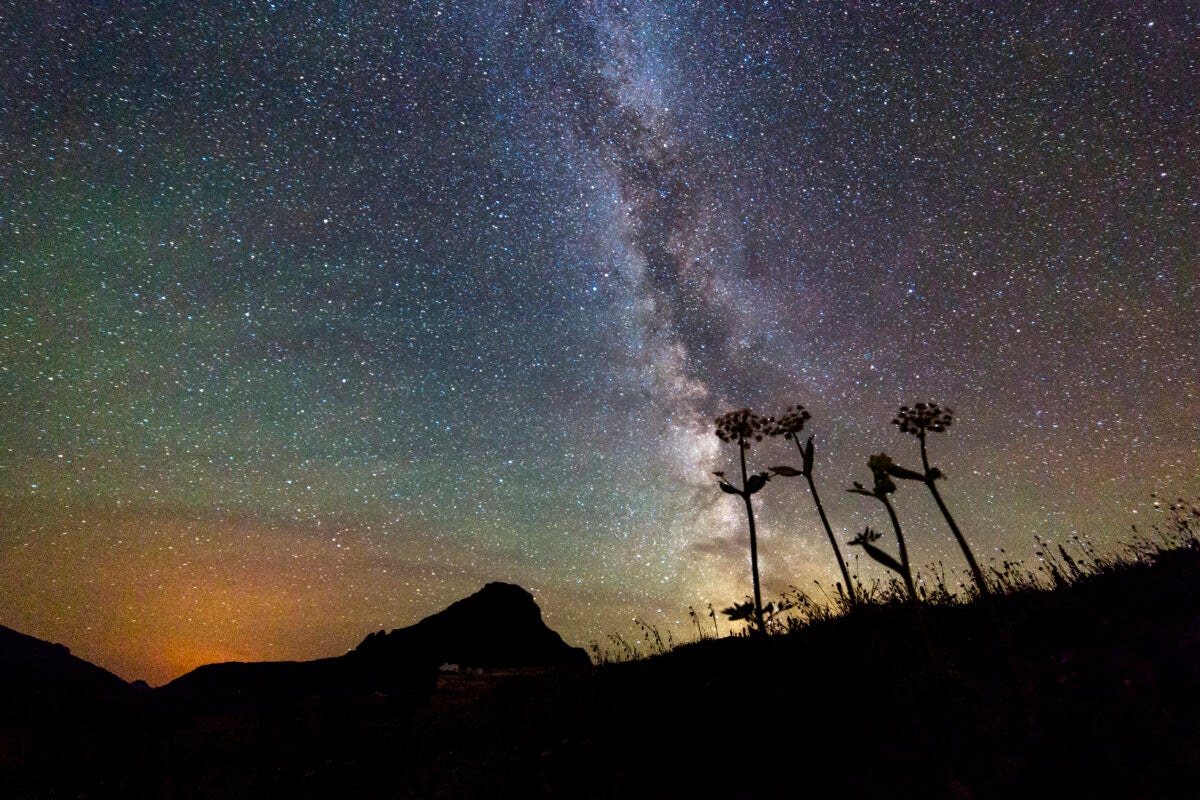
9. What is the universe’s fate?
There are big questions, and then there are big questions. One of the biggest has to be, “What is eventually gonna happen to this thing we call the universe?”
To begin to answer this question, astronomers need to know all about the current physical nature of the universe. This really comes down to which cosmological model we have evidence to support. For a long time, the Big Bang was just one possible model of many.
Fred Hoyle and others pushed the Steady State theory for many years, which proposed a universe that was and will be always expanding, creating matter as it goes to maintain a constant density. Others suggested an oscillating universe that expands outward before collapsing inward and starting over in a series of Big Bangs. In these models, the universe’s fate was a simpler matter, either expanding forever or coming back in on itself in a so-called Big Crunch.
With the discovery of dark energy, the issue became more complex. We can still think of several possible outcomes, however. The first is the so-called Big Rip, in which billions of years from now, the expansion of the cosmos and the growing power of dark energy unbind objects currently held together by gravity. But this is a fairly unlikely scenario.
The likeliest possibility, most cosmologists believe, is the Big Freeze. This would see the universe expanding into an increasingly colder, darker, lonelier cosmos. As stars like the Sun age and die, their remnants will go cold and dark. And as billions and trillions of years pass, any remaining photons will be increasingly redshifted, stretched into wavelengths beyond visual detection. Any vantage point in the cosmos would be left with just a few unimaginably distant dwarf stars, glowing dimly like remote embers, until these, too, wink out.
The universe may have started with a bang, but the likeliest scenario at present is that it will end with a whimper.
10. The meaning of life in the universe
And then there’s perhaps the most fundamental question of all: How did we get here? Some related questions: How did life on Earth originate? How common is life in the universe? Are we alone, or simply isolated from civilizations that exist in huge numbers located across vast gulfs of space, like islands in a cosmic ocean?
From the earliest microbial fossils, we know that life took a foothold on Earth quickly following the Late Heavy Bombardment, about 4 billion years ago. Life on our planet remained very simple for a long, long time. Complex life-forms like us emerged only in the past few millions of years.
The atoms in your body are many, perhaps 7 billion billion billion in the average human. They are the same atoms created in the early universe. Hydrogen and helium were made in the Big Bang, with more massive elements coming from the deaths of stars. The universe is at some level a giant recycling program, and you’re part of it. As another old friend, Carl Sagan, used to say, we are literally star stuff, rearranged into self-replicating cells built into complex, thinking systems.
We know that the conditions for assembling these atoms into complex systems are pretty common throughout the cosmos. Lots of exoplanets that could host life exist relatively near us in the Milky Way. Our galaxy contains something like 400 billion stars. (We don’t know exactly because the most common stars, dwarfs, are dim and hard to see over long distances.) And the cosmos contains at least 100 billion galaxies, perhaps many more.
Conservatively, let’s say that the cosmos contains 10,000 billion billion star systems. We also know, through spectroscopy, that chemistry is uniform throughout the cosmos, and that the stuff of life is plentiful. Do we really think that Earth is the only planet in the whole shooting match to have developed life? Not too likely, a master of understatement would say.
But the distances are vast, and it is almost miraculous enough that we are sentient beings. We can talk about these questions, communicate our feelings and thoughts about the night sky, and gaze up in wonder. How many mysteries of the cosmos will astronomers solve in the coming generations?
Not too many, I hope. Some of what keeps us human is to be outside on a dark night, gazing up at stars and galaxies and simply wondering.