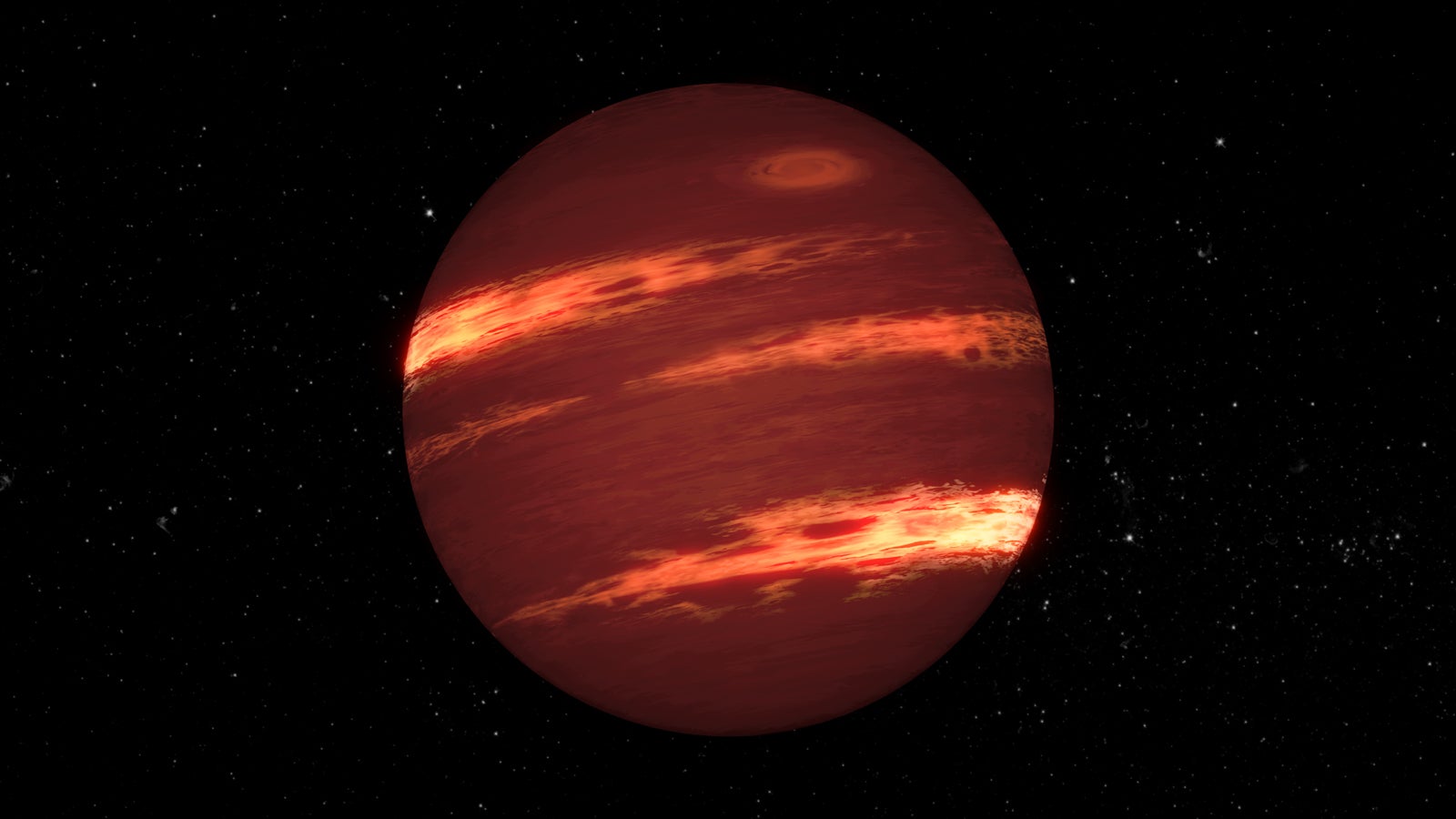
A binary system 1,400 light years away is turning up the heat, and it might help experts better understand the class of exoplanets known as ultra-hot Jupiters — gas giants that are very close to their massive host stars.
The unique system described in a new study published today in Nature Astronomy includes a brown dwarf whose temperature reaches roughly 14,000 degrees Fahrenheit (7,700 degrees Celsius). That makes it hotter than the Sun, whose surface is 10,000 F (5,500 C).
But the brown dwarf’s sweltering temperatures aren’t generated by any internal nuclear reactions of its own: Instead, it orbits very closely to its companion, a white dwarf named WD 0032-317, which is blasting it with radiation. The brown dwarf’s nightside — the side facing away from the white dwarf — is nearly 11,000 degrees Fahrenheit (6,000 degrees Celsius) cooler.
This pair of stars may help scientists learn more about exoplanets that orbit very close to hot, massive stars. The intense bouts of ultraviolet radiation from such stars may cause these planets’ atmospheres to evaporate, and even vaporize its planetary material. But this process is difficult to study.
A white dwarf-brown dwarf system can serve as an analogue for an ultra-hot Jupiter system, one that is much easier to observe. “Jupiter analogues give you an indirect way of studying the atmospheres of giant planets because brown dwarfs should have atmospheres very similar to those of gas giant planets,” says Na’ama Hallakoun, study lead author and observational astrophysicist at the Weizmann Institute of Science in Israel.
Mistaken identity
The WD 0032–317 system was first observed by astronomers conducting a survey of hundreds of white dwarfs in the early 2000s with the Very Large Telescope at Paranal Observatory in Chile. A white dwarf is a star that has reached the final phase of its life — after expanding into a red giant as its fuel runs low, it blows off its outer layers, all that remains is the hot, inert core.
WD 0032–317 was initially flagged as a binary system of two white dwarfs, but when Hallakoun and her team revisited the data, they saw signs that were more telling of a brown dwarf companion.
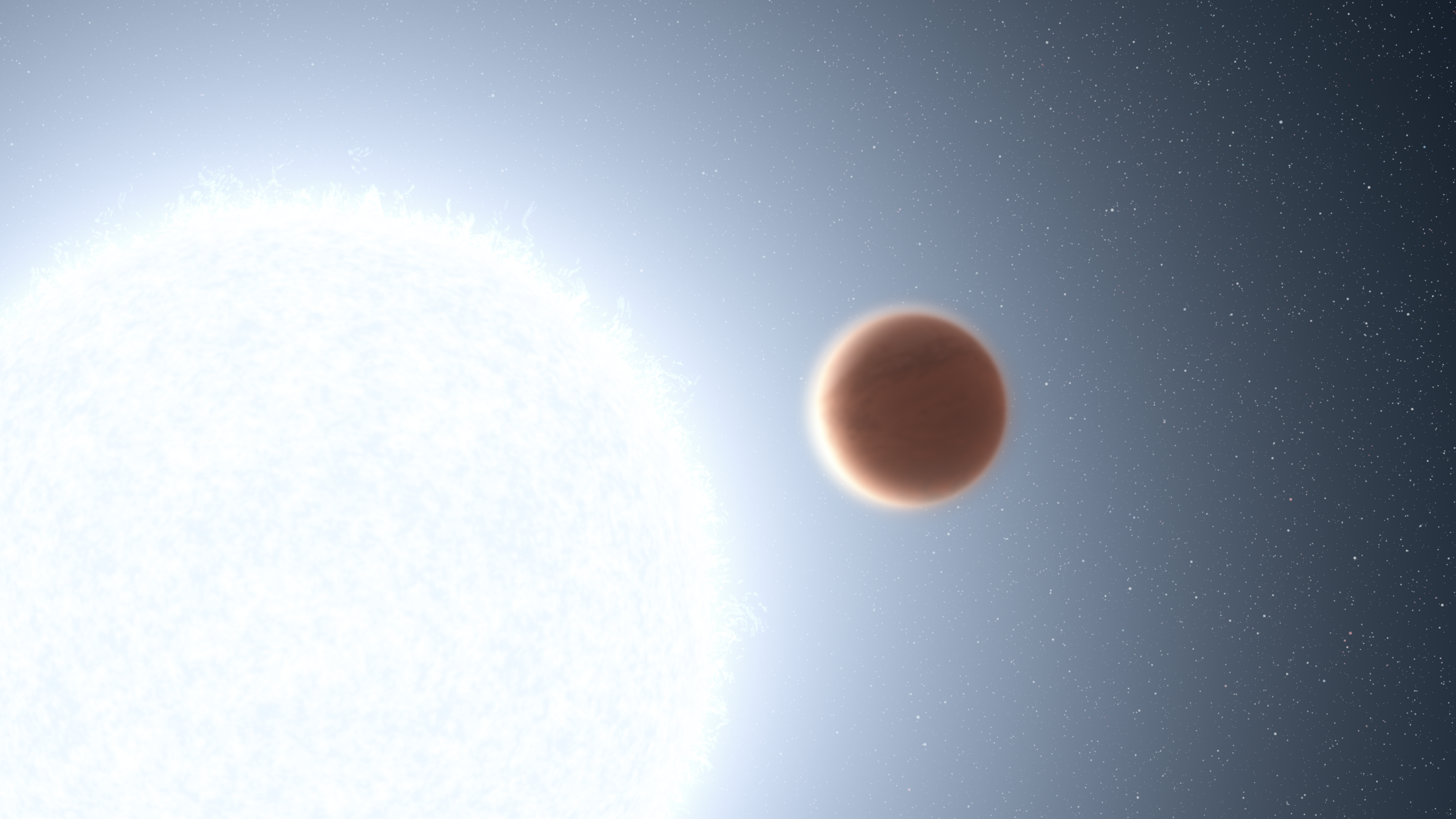
Brown dwarfs are neither planets nor stars, but in-between objects: at least 13 times more massive than Jupiter, but not massive enough to generate the heat and pressure required to fuse hydrogen into helium. For this reason, they are sometimes called failed stars. The brown dwarf may also be one of the largest yet found, weighing in at 75 to 88 times the mass of Jupiter.
In follow-up observations, researchers saw an emission coming from the side always facing the white dwarf. It was originally missed two decades ago because the original observations were taken when the companion’s nightside faced the telescope. In the new data, the brown dwarf’s dayside faced the telescope. “We could see an emission line coming from the irradiated side of the companion,” Hallakoun says. “I was puzzled. My first thought was to think that I had done something wrong during the reduction process of the data.”
An analogue for hot Jupiters
Astronomers would love to observe how planetary atmospheres of hot Jupiters respond to a star’s intense radiation, and watch a planet being so seared by its host star that its molecules begin coming apart. But such ultra-hot Jupiters are hard to observe and detect. Astronomers know of only one such example: KELT-9b, which is so hot it sheds material behind it, mimicking a comet’s tail.
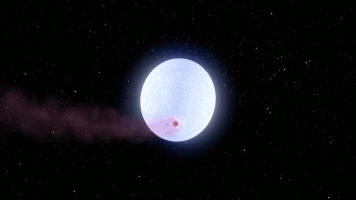
The difficulty of finding ultra-hot Jupiters is in part due to the glare of their large nearby host stars. Complicating matters further is that such stars rotate rapidly and are prone to stellar storms. Astronomers often measure the mass of an exoplanet by measuring the redshift and blueshift of the host star’s spectral lines as the star wobbles due to the exoplanet’s gravitational pull. But when a large star is spinning rapidly and erupting in flares, the rapid movement of the star’s material makes it harder for astronomers to discern the star’s wobble.
For these reasons, astronomers are interested in using brown dwarfs that closely orbit white dwarfs as analogues for ultra-hot Jupiters. The relative sizes of these objects makes the brown dwarf easier to observe: a brown dwarf has roughly the same diameter as a hot Jupiter, but white dwarfs are much smaller than most stars — about the size of Earth. Yet, they can still give off enough residual heat to scorch nearby companions: In the case of WD 0032–317, the amount of extreme ultraviolet radiation that the brown dwarf receives from its white dwarf is 5,600 times higher than that of KELT-9b.
Stellar evolution
Aside from being a model for ultra-hot Jupiters, the WD 0032–317 system also gives scientists a look into the evolution of stars.
Based on models of stellar evolution, the brown dwarf appears to be at least a few billion years old. But the white dwarf is still blazingly hot, indicating it’s only been about 1 million years since it became a white dwarf. What’s more, the white dwarf has a mass of around 0.4 times that of the Sun. According to theory, a white dwarf that small can’t exist on its own — it would take such a low-mass star longer than the age of the universe to reach its white dwarf phase.
Hallakoun suspects that the brown dwarf aided in getting the white dwarf into the state it is in today because they, at one point, shared a common envelope. The common-envelope evolution is a phase in a binary star’s life where two stars or objects orbit inside a shared envelope of gas. In this case, the common envelope developed when the primary star expanded into a red giant, engulfing the brown dwarf.
The brown dwarf may have helped the primary star shed some of its mass and become a white dwarf earlier than expected for a single star, Hallakoun says. “It’s a really young, fast common-envelope system. And we also hope that this system and other such systems will help us better constrain the theoretical models we have to explain binary motions.”