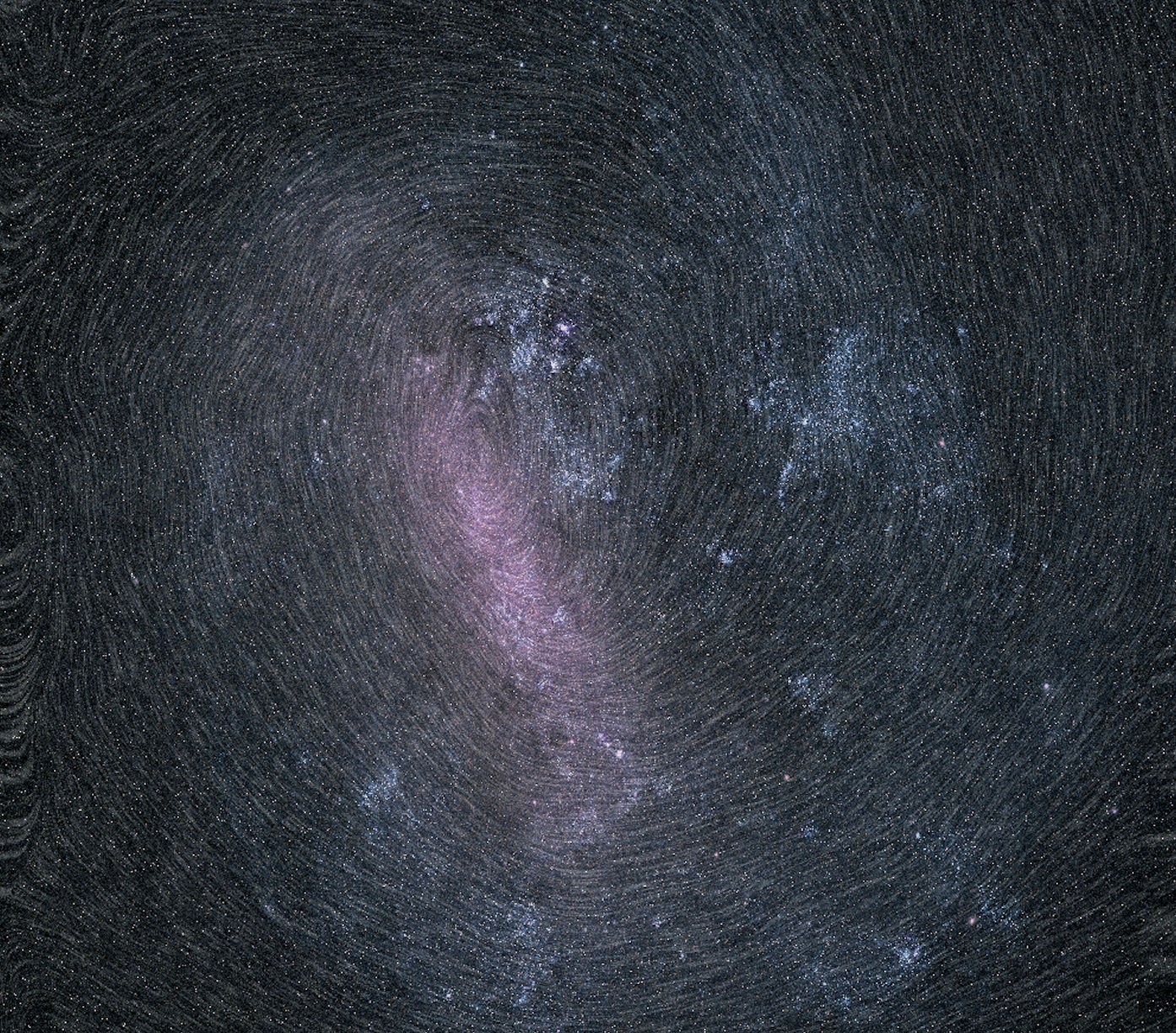
Our planet is in constant flux: Weather, erosion, and plate tectonics mean Earth is never the same from one moment to the next. This is in stark contrast to the firmament above, a seeming paragon of stability whose countless stars are reminiscent of shimmering dots securely glued to assigned points on a rotating domed ceiling. In this realm only the Sun, Moon and five naked-eye planets appear to move independently of their stellar comrades in the otherwise fixed astral framework.
Until only a few centuries ago this picture of rigidly fixed heavenly bodies circling a centralized Earth was firmly entrenched. Little by little, though, through the efforts of women and men worldwide, illusions of cosmic steadfastness vanished. Over the years we learned that individual stars budge and waver slightly under several subtle influences. Some are periodic, others cumulative, and all require a careful eye to observe.
Slow and steady
Probably the easiest to comprehend is proper motion: the observed rate, as perceived from Earth, of a nearby star sliding along against the background of a seemingly motionless celestial expanse. This motion is mostly apparent: while stars do cruise about on their own paths, the motion is far more noticeable with closer stars, and we measure it according to how dramatic the change appears to us on Earth.
Imagine you are driving down a one-way highway. Your car is the Sun. Most of the other cars (stars) will be traveling at more or less the same speed, appearing to travel in lock step with you. This is because we’re all moving with the galaxy’s flow, in a sense following the rules of the road. Those far in the distance remain in the distance, and it’s difficult to tell whether they’re getting closer or farther from you, or even when they change lanes. But for those near you, even a small change in speed is readily apparent, and it is very obvious when a car changes lanes near you.
In the same way, proper motion is a combination of stars’ actual movements and their proximity to us.
We measure proper motion in arcseconds per year where 60″ (arcseconds) make an arcminute, 60′ (arcminutes) make a degree, and the usual 360° describe a full circle. Proper motion is given in both right ascension and declination — which correspond to longitude and latitude here on Earth — in the two-dimensional sky we know and easily understand.
That third dimension – radial distance – is far trickier to measure, and left to other kinds of observations.
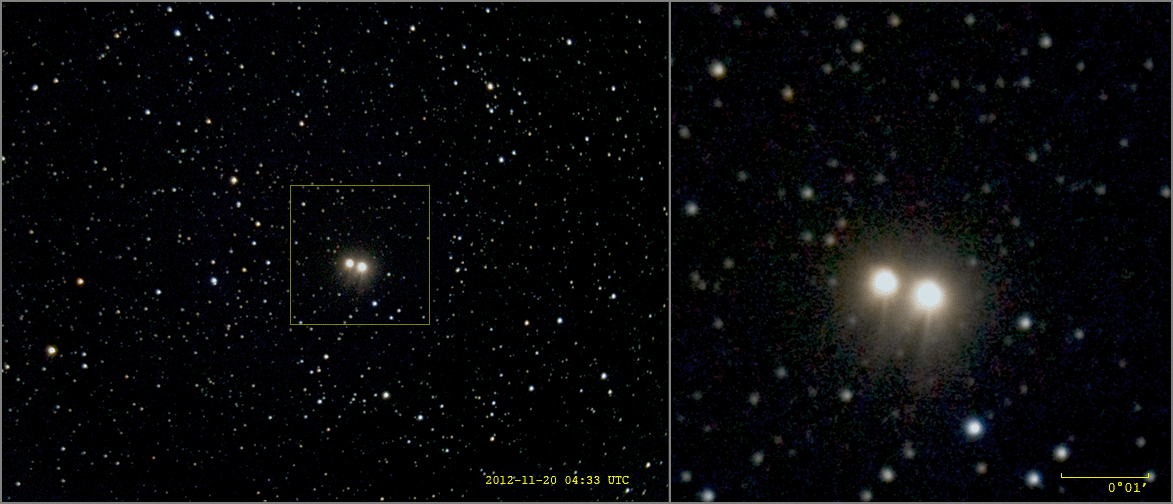
Barnard’s Star in the constellation Ophiuchus exhibits the greatest proper motion of all, 10.3″ annually, sufficient to bridge the diameter of the Full Moon in 175 years. This is trivial in terms of everyday notice and indicative of the slow pace of the extraterrestrial domain. Barnard’s is the fourth nearest star to us but despite its proximity, it requires optical aid. While appearing on photographs from 1888 and 1890, its progression went unnoticed until its “official” discovery at Yerkes Observatory in Wisconsin in 1916 by the eminent American astronomer E. E. Barnard.
Barnard’s Star is known for its quick path across the constellations. But for most stars, it takes centuries or millennia for the tiny motions to add up enough to change how we see the constellations from one year to the next.
Forward and backward
A second aspect of stellar meandering is “parallax”, the shift in position of an object with respect to a remote background when viewed from two separate vantages. The simplest example can be seen by extending a hand, raising a thumb, and alternately opening and closing your left and right eyes. Your thumb will appear to shift position relative to the background, but the only thing “moving” is your point of view.
And while proper motion is only partially dependent on distance, parallax is a direct measurement — the closer the star, the larger the parallax. Stellar parallax is most pronounced at six-month intervals when Earth is on opposite sides of our nearly perfect circular orbit around the sun. Parallax is a much smaller effect than proper motion, when comparing stars at the same distance.
Compared to its proper motion, 3.5″ annually, the parallax of the Alpha Centauri system is a paltry 0.7687″ (+/- .003″), the greatest among all the stars, corresponding to a distance of 4.3 light-years, the closest of all the stars.
Parallax reverses itself every six months, so it doesn’t accumulate any change the way proper motion does. Parallax merely shifts the stars back and forth slightly.
German astronomer Friedrich Bessel was the first to confirm stellar parallax when, in 1838, he detected a shift for the dim naked eye star 61 Cygni, leading to the first distance measure of any object outside the solar system.
Throughout all of ancient and medieval history, up until Bessel’s impressive achievement, stellar parallax eluded observers, falsely implying that the Earth stood still. Long before it was ever observed in astronomy, Earth-bound scientists figured out that if we revolve around the Sun, the constellations should swell and shrink as we approach and recede from them. The absence of such pulsations reinforced the case for our planet’s immobility.
It was the immense distance between the solar system and the stars, compared to the much lesser Earth-sun separation, that fooled observers for centuries. They simply couldn’t fathom the hugeness of the universe.
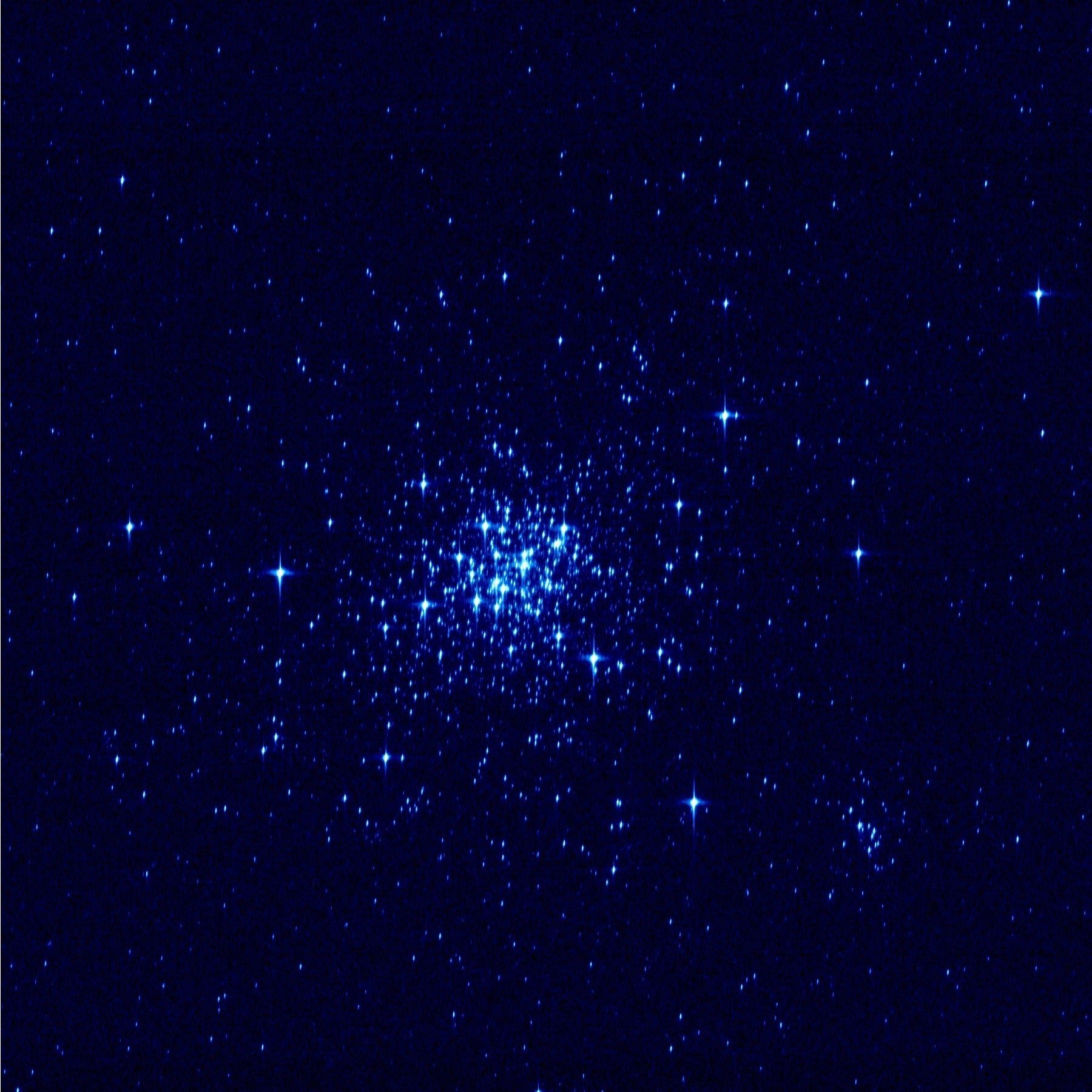
Twinkle, twinkle
Under ideal night sky conditions, stars appear as point sources in an exact location – pinpricks of neatly identifiable light. However, with Earth’s inherently unsteady atmosphere and the consequent swelling and instability of stellar images, exact addresses can be difficult to ascertain. Accordingly, ground based measurements of parallax are accurate to no more than about three hundred light-years, covering only a fragment of our galaxy. Instruments such as the Gaia space telescope extend the range to tens of thousands of light-years.
Almost everyone has seen refraction – such as when a straw appears to bend in glass of water. Similarly, Earth’s atmosphere refracts light from celestial objects. The effect is near zero at the zenith (directly overhead) and increases toward the horizon where it can reach 35.4′, depending on atmospheric pressure and temperature. A major outcome of this is the perceived early rising and delayed setting of the Sun — and other objects in the sky — giving us a few more minutes of daytime every morning and evening than we would have if we were airless.
Another effect is the very pronounced twinkling of stars close to the horizon, as their light traverses more atmosphere, thereby undergoing more instability. The Sun, the Moon, and planets, all having measurable sizes, experience different impacts. Their images dance, distort and discolor when rising or setting under a less than steady sky.
As we have seen, things are not always as they seem. Unruly situations and tricky subtleties infiltrate the sky. When we look up at the stars in an attempt to unravel their uncertainties, they mischievously smile down at us. It takes rigorous surveillance for humans to notice their modest migrations, which gives us all reason to continue gazing skyward.