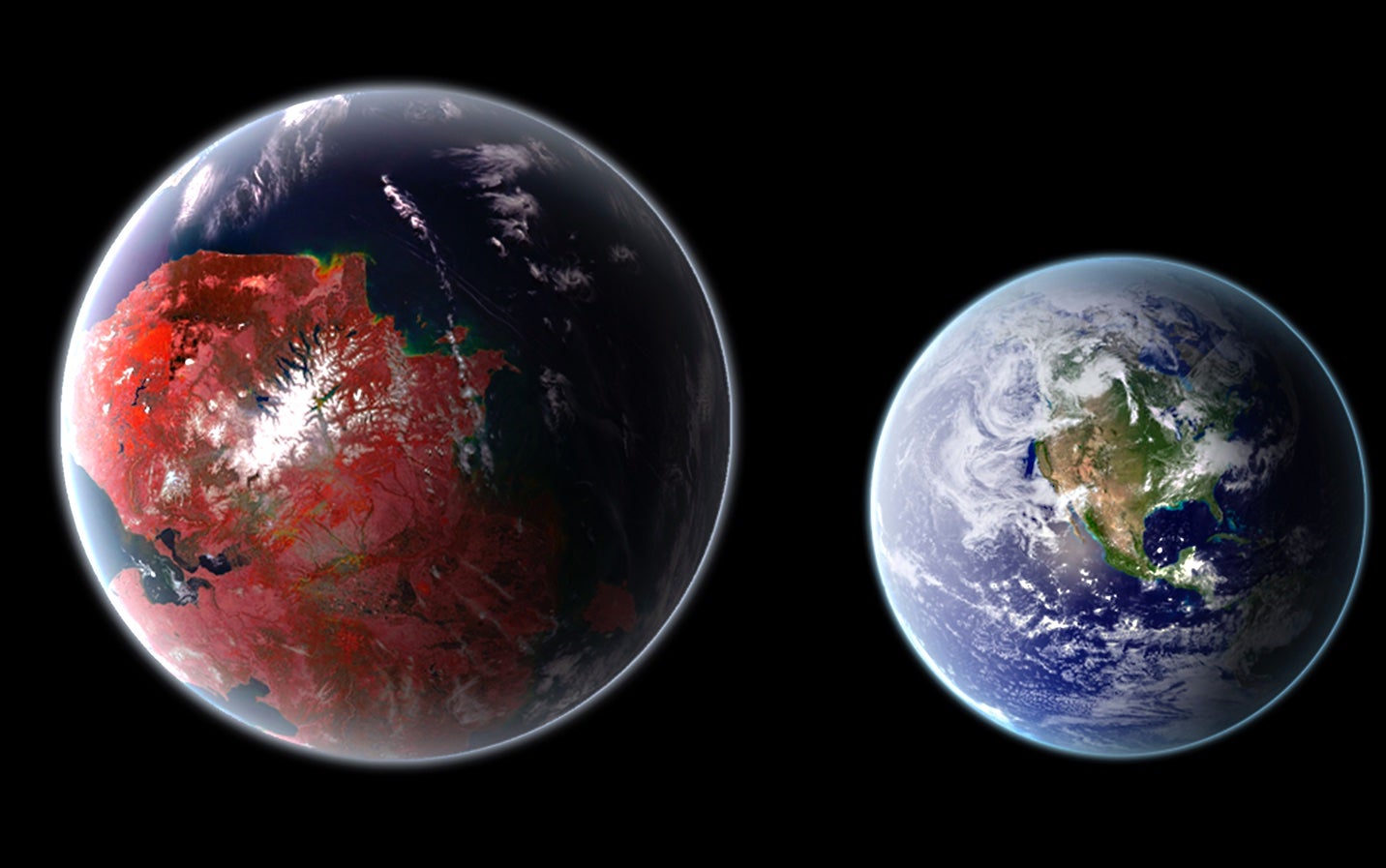
Humans have wondered about life in the universe since antiquity. Early Greek philosophers argued that the cosmos contained “a plurality of worlds.” Today we know that exoplanetary systems are indeed ubiquitous, but the question now is: How common are planets that can support life?
In 2000, paleontologist Peter Ward and astronomer Donald Brownlee of the University of Washington in Seattle penned a controversial book, Rare Earth: Why Complex Life Is Uncommon in the Universe (Copernicus). In it, they propose that Earth is an unusual world where complex life-forms developed over an extended period. They further assert that though simple, unicellular life may be abundant in the universe, complex life must be exceedingly rare.
In sharp contrast, noted Harvard-Smithsonian Center for Astrophysics astrophysicist Avi Loeb passionately argues in his book Extraterrestrial: The First Sign of Intelligent Life Beyond Earth (Mariner Books, 2021): “Given the ubiquity of habitable planets, it is the height of arrogance to conclude that we are unique.”
The debate about habitability and life in the universe remains wide open. In the past, astronomers spoke of finding “Goldilocks worlds” — planets in the habitable zone (HZ) of their stars, where the temperature was just right for liquid water on the surface. But the past two decades of research have shown that just because a planet lies in the HZ doesn’t mean it’s necessarily habitable.
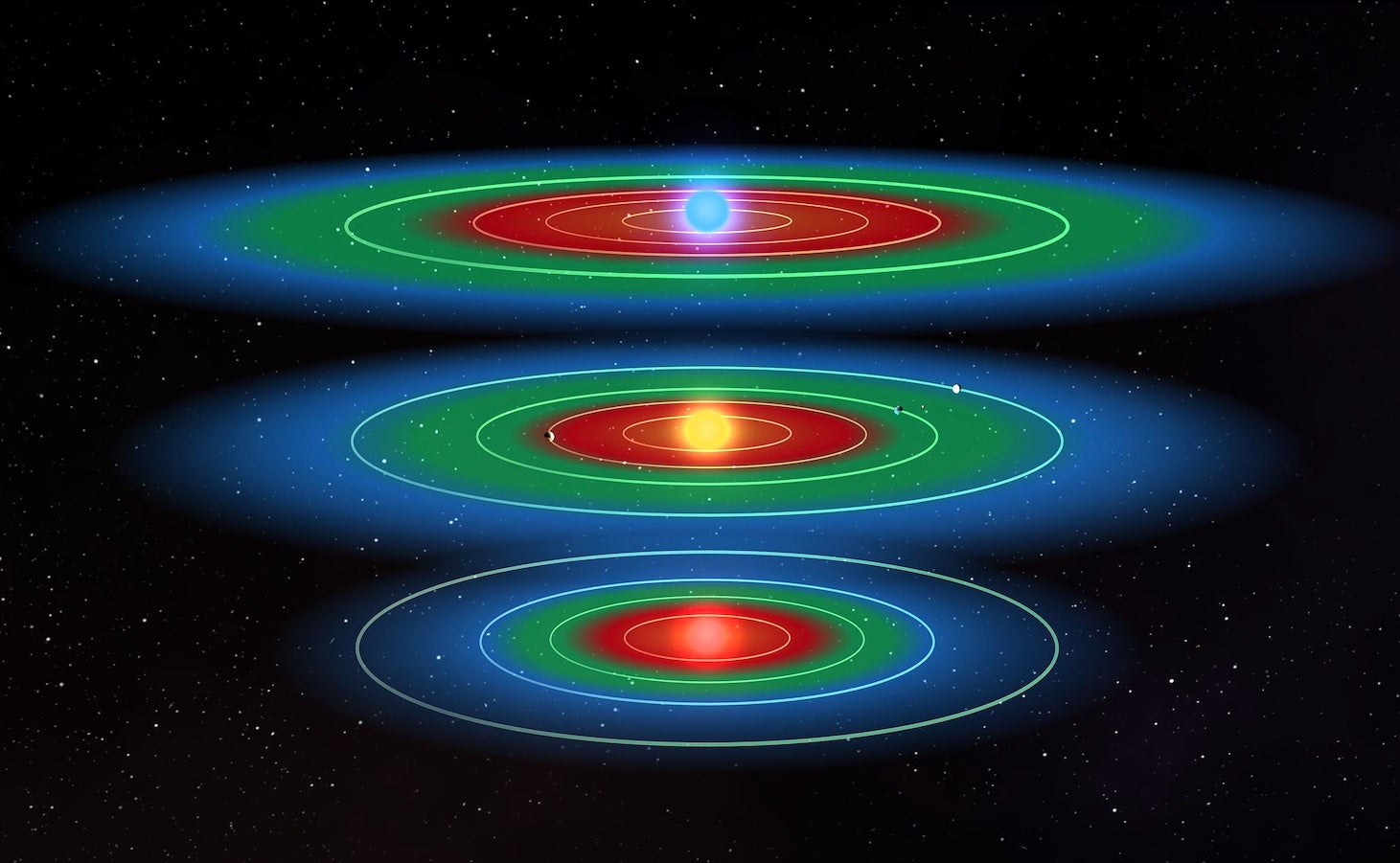
The Goldilocks zone
In Rare Earth, Ward and Brownlee suggest that for complex life to evolve, several requirements seem essential: a terrestrial-type planet of appropriate size with a stable orbit, inside the habitable zone of a stable star. In addition, the planet needs the right atmosphere, plate tectonics, and a large moon, and must reside within the right type of system. If most of these attributes, they argue, are not met or in place for several billion years, complex life is unlikely to appear.
Scientists recognize two types of habitable zones: stellar and galactic. The first is the orbital region around a star where a planet can support liquid water on its surface.
The second type is the galactic habitable zone (GHZ), encompassing a region in the Milky Way in which a star meets other criteria for supporting complex life. This region is far from the galactic center, where star density is much higher than in the Sun’s location. Hence, Earth is not as exposed to potentially deadly supernovae and gamma-ray bursts detrimental to complex life.
The concept of the stellar habitable zone has changed since its introduction by astrophysicist Su-Shu Huang in 1959. He argued that the best candidates for hosting planets within habitable zones are probably F, G, and K stars, which range from about 0.6 to 1.6 times the mass of the Sun. While this assertion has pretty much stood the test of time, our Earth-centric perspective of habitability has since broadened significantly.
As radio astronomer Alan Bridle of the National Radio Astronomy Observatory in Charlottesville, Virginia, succinctly puts it: “Habitable by what?” He asks, “Supposing sentient creatures had happily evolved in an ocean under the ice of Enceladus or Europa, would they be mystified by arguments that the HZ for water-dependent life does not extend as far as Mars?”
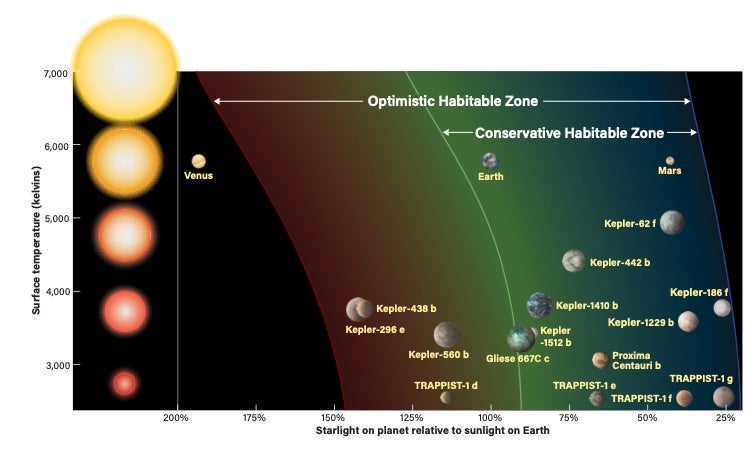
The case for Rare Earth
So, are Goldilocks worlds (like Earth) capable of fostering technologically advanced life-forms rare in our galaxy? When Rare Earth was published, only about 100 exoplanets had been found, most of which were easy-to-detect gas giants. What is the state of affairs two decades and some 5,000 planets later?
Ward and Brownlee argue that the right type of star system for hosting complex life is one like ours, with a single main sequence star, rocky planets in its HZ, and an outlying gas giant like Jupiter. The Sun is G spectral class, which comprises about 9 percent of hydrogen-burning stars in the Milky Way. M-class dwarf stars are the most common and longest-lived stars. However, they seem less suitable because they bombard their planets with radiation from stellar flares.
Also, because the HZs around M dwarfs are relatively close in, planets there are subjected to tidal forces that lock their rotation, leaving one side hot and one side cold. Solar-type stars appear most suitable because their luminosity doesn’t vary as much, and they still have relatively long lives — 4 billion to 10 billion years. Higher-mass stars are hotter and shorter lived.
Other key components in the supposition that Earths are rare are the presence of Jupiter, our relatively large Moon, and plate tectonics. Because Jupiter’s gravitational influence deflected asteroids and comets away from the early inner solar system, it lowered the frequency of Earth impacts and allowed life to thrive and evolve. And the Moon plays a crucial role by keeping our planet’s tilt around 23°, which ensures seasonal cycles and ocean tides are not too extreme. Our large satellite also generated tidal forces that induced plate tectonics in Earth’s crust. Those processes are thought to allow steady evolutionary change, allowing life to diversify and grow more complex.
Also deemed crucial are liquid water and our planet’s magnetic field. Water, as we know, is vital for life on Earth. And our planet’s strong magnetic field shields its surface from harmful solar radiation and cosmic rays, so it likely facilitated the genesis of complex life.
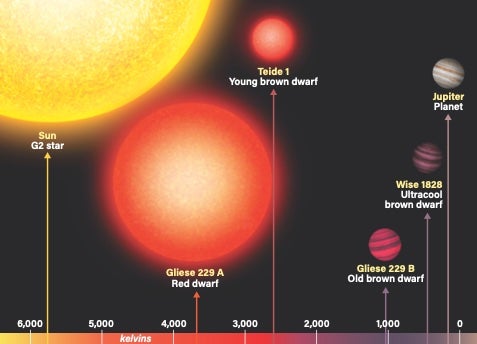
The case against Rare Earth
Despite the arguments outlined above, most researchers today either withhold judgment or disagree with notions that Earth is anomalous. The consensus is that we simply do not have enough data to understand how Earth-like planets form and evolve over time.
Although astronomers have detected more than 5,000 exoplanets so far, the bulk of them come from NASA’s Kepler mission. There are limitations to this sample: Kepler preferentially detected relatively large planets close to their stars, while rocky planets were less likely to be spotted. That skews the exoplanet statistics and likely undercounts the number of rocky worlds.
Researchers have also questioned some of the major tenets of the Rare Earth hypothesis, like the scarcity of moons like Earth’s. As University of Arizona astronomer Chris Impey points out in The Living Cosmos (Random House, 2007), even if the Moon is pivotal for life on Earth, there is no a priori reason that such moons can’t form around other Earth-like planets.
Opponents of the Rare Earth hypothesis have also suggested that the influence of a jovian planet in the solar system is far more uncertain than Rare Earth asserts. In a 2008 series of papers titled “Jupiter: Friend or Foe?” Jonti Horner of the University of Southern Queensland and the late Barrie Jones argue that rather than shielding Earth against external impactors, Jupiter had the opposite effect. Likewise, in a 2016 paper, planetary physicist Kevin Grazier reported computer simulations that found that Jupiter tended to keep comets’ orbits from migrating outward, increasing the odds that they would collide with Earth. However, Grazier argues, this benefited life’s development in a different way — by delivering “life-enabling volatiles including carbon to the terrestrial planets.”
Are we exceptional —or the first?
Should we learn that planets like ours are indeed infrequent or unusual as Rare Earth proponents maintain, where would that leave us? It has taken more than 4 billion years for Homo sapiens to appear. If such timelines are universal, then perhaps we are the first in the galaxy to reach that status.
This would explain the paradox posed by physicist Enrico Fermi in 1950: If intelligent life is common in the universe, then where is everybody? It’s possible that intelligent civilizations are either rare or just emerging in the Milky Way and our searches should adjust for that possibility.
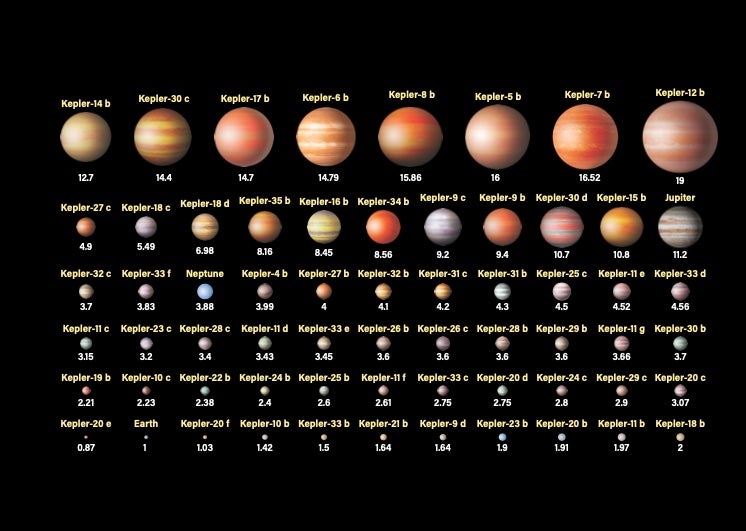
Altering our mindset
The Rare Earth hypothesis leaves much room for speculation. We may have to rethink key factors. The first is the standard notion of habitability that centers around liquid water and a solid planetary surface. We must expand this to include the prospect that life may thrive underground or in oceans under ice, as well as on moons orbiting planets.
Second, our concept of life is by necessity Earth-centered, but that is clearly too narrow. Humans thrive in an aerobic environment, but several species of roundworms and crustaceans thrive in anoxic marine sediments, showing that aerobic respiration is not indispensable for animals even on Earth. That is likely true on other worlds and raises the question of alternative life-forms.
Third, we still need stronger data on how common Earth-like planets are in our galaxy. Presently only a handful of exoplanets fit the known criteria to be able to hold an Earth-like atmosphere. In a 2021 study, Giovanni Covone and colleagues at the University of Naples analyzed the efficiency of photosynthesis on potential Earth-like planets in the HZ. “This study puts strong constraints on the parameter space for complex life,” Covone said in a statement. “[U]nfortunately it appears that the ‘sweet spot’ for hosting a rich Earth-like biosphere is not so wide.” However, a 2020 NASA study suggested that about half of Sun-like stars could host potentially habitable rocky planets. Obviously, our picture is far from complete.
Two additional factors regarding intelligence and survival of advanced civilizations are the anthropic principle and the Great Filter. First outlined in 1970, the anthropic principle attempts to define the credibility of our observations of the universe, given that we can only exist in this particular universe. Our existence would not be possible if the laws of physics were incompatible with the development of sentient life. This reasoning has been used to advance the notion that the universe is somehow fine-tuned for life.
The Great Filter, in a nutshell, posits that we might be alone because there are so many improbable steps in the evolution of higher intelligence that it is unlikely to occur multiple times. Coupled with our potential to self-destruct, perhaps that’s why technologically advanced civilizations are rare or don’t last long, thereby explaining the Fermi paradox.
The debate rages on
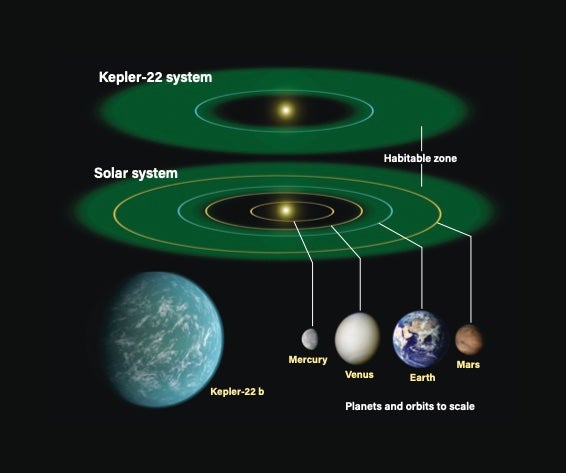
In their soon-to-be-published new book, The Rare Earth Hypothesis, Ward and Brownlee double down on their thesis with what they consider even stronger supporting evidence. They posit that “from what we know now, rare might not be a restrictive enough word for describing the frequency of complex life in the Cosmos.” Their conclusion is based on comparisons of most of the exoplanetary systems so far characterized and that “no potentially habitable planet will remain so for long in almost every case without several crucial aspects.” Foremost is an ability to maintain a temperature allowing the existence of liquid water for almost unimaginable periods of time — which, they maintain, is perhaps the most important aspect of life-supporting planetary systems. The authors also state that had a body the size of Comet Hale-Bopp (C/1995 O1) struck us, “there probably would not be a single microbe alive on Earth right now, let alone animals.”
In a paper titled “The Astrobiological Weak and Strong Limits for Intelligent Life,” astronomers Tom Westby and Christopher Conselice of the University of Nottingham attempt to estimate how many communicating civilizations might exist in our galaxy at any given time. Deriving models that assume an average lifetime of such civilizations as 100 to 200 years (based on our own ascent to radio communications capability), they conclude that, optimistically, there might be as many as 928 such civilizations within some 3,300 light-years. More pessimistically, there might only be 36 within 17,000 light-years. Clearly, in our effort to assess whether Earth, and by extension humanity, is exceptional in the cosmos, no firm conclusions are possible.
Only time will tell, although current evidence strongly implies that a Star Trek depiction of abundant civilizations in the galaxy must remain in the realm of science fiction.