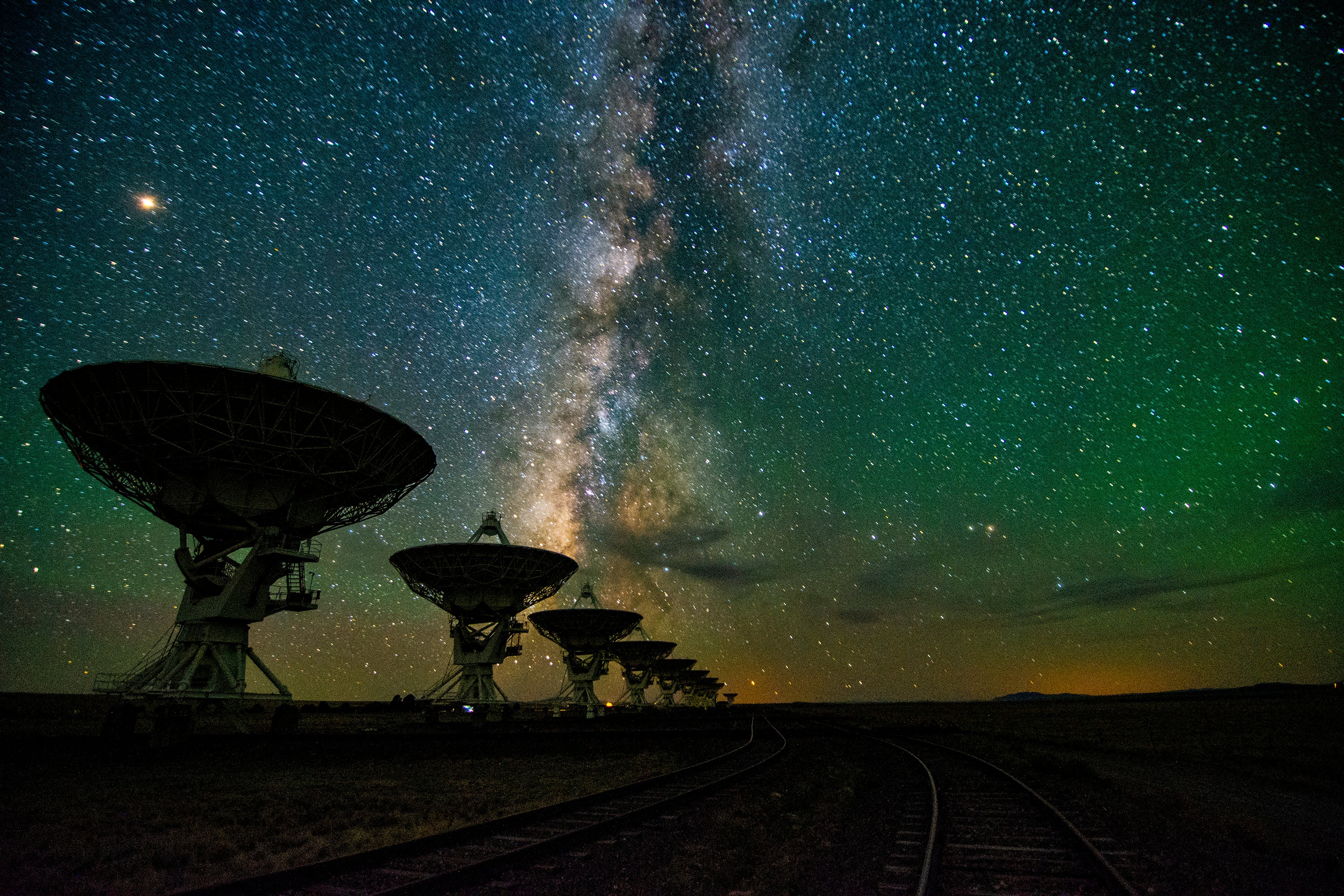
I have just replaced Albert Einstein (a.d. 1879–1955) with Pythagoras (570–490 b.c.) as the one person, living or dead, with whom I would like to have dinner.
That is quite a turnaround for me, since his Pythagorean theorem was the reason I failed my 10th-grade geometry class. It was enough to scare me away for years — and keep me from learning about a man considered a giant in the early studies of math, music, philosophy, and astronomy.
But since my main two hobbies in high school were astronomy and music, I wish my teacher would have shared with me that Pythagoras is the man who coined the term cosmos and was instrumental in the development of understanding the physics of musical sound.
The overtone series
Among his many accomplishments, Pythagoras is credited with the discovery and understanding of the overtone series — the series of frequencies contained in most musical notes, creating the distinctive sound you hear. Before I was introduced to the overtone series, I had taken musical pitch at face value without knowing it takes a series of mathematically related tones to create what I heard as familiar and pleasing. After I learned about overtones, I never listened to music in the same way.
In his 1619 book Harmonices Mundi (Harmony of the World), Johannes Kepler (1571–1630) describes Pythagoras passing through a smithy and noticing that hammers of different sizes created sounds with different pitches on the anvil. Pythagoras then moved from hammers to strings, observing how different lengths of string oscillated at different frequencies, producing various tones. (Today, this exact story is considered apocryphal; likely Pythagoras made this discovery using only strings, no smithy required.)
Regardless of the chain of events, Pythagoras discovered that when a musician plays a note and causes a musical instrument to vibrate, the root note that we hear (called the fundamental or first harmonic) is also accompanied by a series of higher frequencies. These occur naturally in a strict mathematical sequence and depending on the strength of the overtones that occur above the fundamental, produces the tone and character of an instrument — which is also referred to as timbre. Without those overtones, the result would be a very unsatisfying sine wave. Pythagoras identified these overtones as harmonic frequencies using mathematical ratios. One-half of the fundamental (1:1) creates an octave (2:1) above the original pitch, with the third overtone — the perfect fifth — vibrating at three times that of the fundamental above the second overtone (3:2), and so on. This sequence continues into ever higher frequencies, eventually beyond what the human ear can detect. An instrument’s unique voice comes from the fact that each uses a different combination of frequency strengths to produce the same note.
In other words, the harmonics of the overtone series deliver rich tonal complexities. However, their existence is hidden within the sound we hear in the same way that light we perceive as white combines all the wavelengths of the visible spectrum.
Heavenly motions
Where does this musical finding intersect with the cosmos? Pythagoras took his earthly discovery and sought to connect it to the workings of the sky above. Building on his realization that “there is geometry in the humming of the strings,” Pythagoras believed “there is music in the spacing of the spheres” of the planets visible in the sky.
This belief had long-lasting implications: Sixteen centuries later, music, math, and astronomy were complementary studies. Seeking connection between the physical world and the cosmos, Galileo Galilei (1564–1642) stated: “Mathematics is the alphabet with which God has written the universe.”
Around the same time, Kepler was attempting to expand Pythagoras’ concepts of mathematical ratios and musical intervals (including overtones) in Harmonices Mundi, reflecting what he believed was God’s design and what the soul naturally senses. “The heavenly motions are nothing but a continuous song for several voices, to be perceived by the intellect, not by the ear,” he wrote. His intention was to illuminate how the six known planets of that time orbit the Sun and produce distinctive tones relative to their distance and in harmonic relationship with each other — the “music of the spheres.”
NASA’s A Universe of Sound project is an ongoing program to sonify astronomical image data taken at multiple wavelengths. Targets include the galactic center (above), the Cassiopeia A supernova remnant, and the Pillars of Creation . You can explore the available sonifications here.
Sonification
Enriching the exploration between sound and space is the recent increased interest in sonification — the process of converting data into sound. While abstract for some, it creates a new world of imagery for blind or visually impaired individuals interested in or working in the sciences.
Sound engineers and astronomers are now collaborating to create a vocabulary using musical terms such as timbre, tone, and pitch, associating them with spatial imaging. Imagine an astronomical image as a field of complex data points containing information such as brightness and wavelength, which taken together can have a host of other visual descriptors often taken for granted by fully sighted people. Via sonification, these points become audio representations, allowing the visually impaired to experience the cosmos — and sighted individuals to experience visual and other information in an entirely new way.
Listening to the universe
Pythagoras, Kepler, and others may have professed a musical connection to the cosmos, but current technology provides definitive ways in which sound can show us the stars. In a sense, the rhythmic waves, pulses, and bursts we receive from space are all elements of the soundtrack to our universe.
For instance, in 1964, Arno Penzias and Robert Wilson picked up what we now know to be the cosmic microwave background (CMB) radiation, the echo from the Big Bang of 13.8 billion years ago. What first appeared to them as background noise ultimately provided evidence for much of modern cosmology. I find it noteworthy that the term itself, Big Bang, implies an explosion of sound, although that could not have happened because what we define as sound is created by vibrating air molecules. This is why screaming in the vacuum of space will not get you any attention: There is no air to transmit the vibration and create sound.
But although the limitations of the human ear require us to rely on processing technology to make that which is inaudible audible, we can still “hear” the extraordinarily low frequencies and respective overtones of the early universe, as captured by satellites such as Planck, through the CMB. The resulting sound is nothing short of mesmerizing.
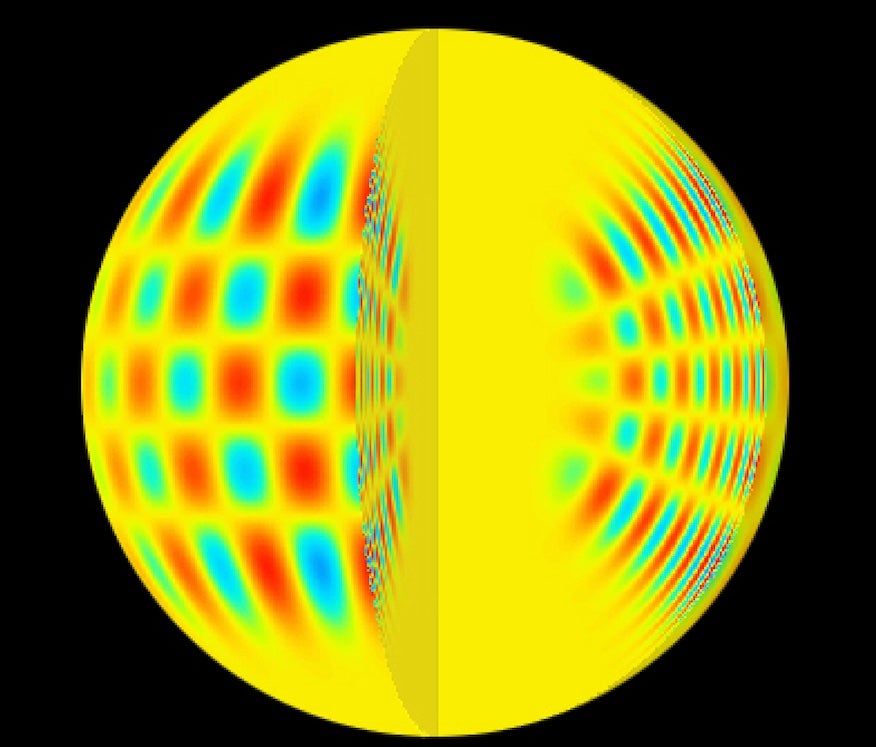
Our ears are further limited as they can only detect frequencies between 20 and 20,000 Hertz, which puts many natural vibrations outside of our hearing range. Our Earth generates audible and inaudible sounds from deep within the planet, down to background hums as deep as 10,000 times lower than the ear can hear.
High above, electromagnetic waves propagating through the inner region of Earth’s magnetosphere, called the plasmasphere, create their own type of music. In this space above our planet, waves such as whistler-mode waves, chorus waves, and plasmaspheric hiss waves are associated with everything from the tug of the Moon to lightning strikes. (Lightning also creates waves that have been seen to bounce and “ring” through the ionosphere.)
The plasma there is far too thin for its vibrations to be able to tickle our eardrums. But, as with the CMB, these electromagnetic waves can be recorded by antennas and converted into sound. For instance, lightning causes electrons to oscillate in waves that, when played through speakers, sound like whistles — hence the name whistler-mode waves. It’s worth noting that transforming such waves into audible sound is akin to the way visual astronomers collect light at frequencies our eyes can’t see — say, infrared light using the James Webb Space Telescope — and transform it into a visual image.
These waves are not unique to Earth. Voyager 1 first recorded whistler-mode waves associated with lightning in Jupiter’s plasmasphere in 1979. Now referred to as jovian whistlers, they provide proof of otherworldly lightning and share with us a small taste of sound from the largest planet in our solar system. Both Voyager spacecraft have also now passed through the heliopause — the boundary of our solar system where the solar wind collides with the surrounding interstellar plasma — and have recorded many types of oscillating plasma waves. Ironically, as we listen to interstellar space and the phenomena that shape it, from under-lying galactic rotation to supernovae and smaller solar eruptions, the Voyagers carry a representative sample of Earth’s natural sounds and musical compositions in the form of the Golden Record produced by NASA and Carl Sagan.
Many stars oscillate in numerous ways as well, from hydrogen-burning main sequence stars like our Sun to pulsating variable stars to quaking neutron stars. Some oscillations are due to turbulent plasma trapped in stars’ upper layers; others come with changes brought on by age. Starquakes and pulsations can produce variations in a star’s brightness, and observations of these oscillations form the basis for the fields of helioseismology and asteroseismology. Such studies allow a further understanding of the inner structure and workings of stars at every stage of their evolution. If we accept that the physics of sound Pythagoras discovered holds constant, then it is plausible that all these events produce pitches, overtones, and harmonics with frequencies that can be converted into the audible range.
Even catastrophic events can yield auditory treasure. A study published earlier this year in Nature revealed that some gamma-ray bursts (GRBs) contain more than just noise. GRBs that occur when two neutron stars collide can create short-lived hypermassive neutron stars (HMNS), the fastest spinning stars in the universe. They are difficult to study, lasting only seconds before collapsing into a black hole. In the paper, astronomers reported they were able to recognize the presence of an HMNS by the frequency at which the staccato blast of gamma rays flickered. The trick is that the oscillations of HMNSs lack a consistent pitch; instead, they produce a variety of so-called quasi-periodic oscillations around a peak frequency, adding clamor to our celestial orchestra.
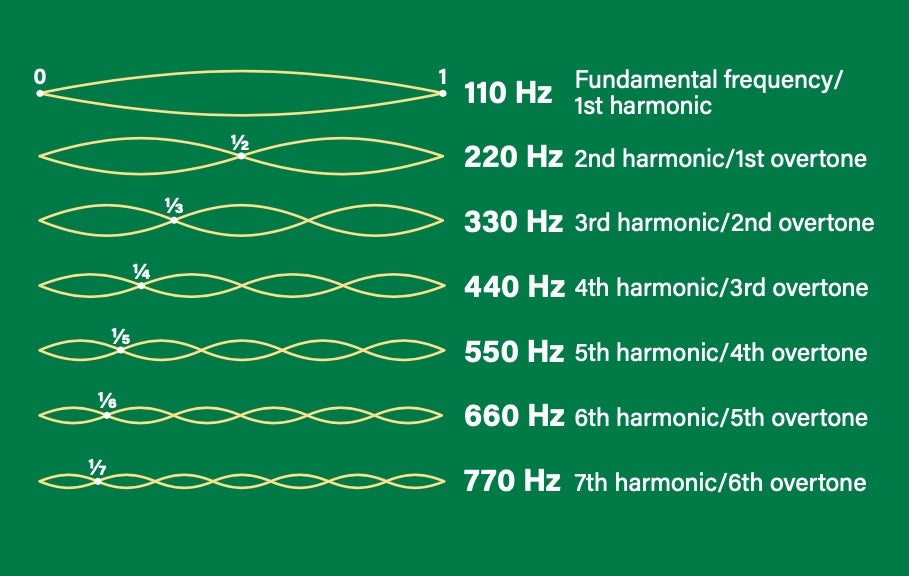
What is music?
I imagine the future may hold similarly difficult-to-define patterns that will need more complex deciphering. In the meantime, the variety of identifiable signals we receive can be recorded, processed, resynthesized, and brought into the audible range. With varying rhythm and amplitude, some pitches and sounds rise and fall, while others remain constant. They can be reflective of lions roaring, a heart beating, or familiar ever-present white noise. More tonal results have been referred to as birds chirping or flocking, early video game sound effects, or ascending and descending vocal glissandos.
None of the samples we obtain from space may be music as most of society has come to define it. But is there a definitive definition of music? Today’s contemporary music would probably be unidentifiable to the ears of the first singers of Gregorian chant. And consider “4′ 33″” by the celebrated 20th-century composer John Cage, who believed the three-movement composition his most important work. It is four minutes and 33 seconds in which the performer sits in silence — which provokes the question of whether any and every auditory experience can be regarded as musical.
Is it possible that the breathtaking sight of a globular cluster with exoplanets rotating around their suns also produces yet-to-be-captured sounds? Could galactic groups generate collective waves that create a unique cosmic symphony? Maybe this would be the true music of the spheres. Would we perceive what we hear as a cacophonous jungle filled with alien, unconnected sounds? To some, yes. But that same term — cacophony — has also been used to describe the sound created when multiple piano keys are simultaneously played as closely as possible. In composition, this is referred to as a cluster chord, which sounds like noise to many but is nonetheless 100 percent musical.
Twenty-four centuries after Pythagoras died, Danish composer Rued Langgaard wrote a groundbreaking orchestral homage in his “Music of the Spheres.” More than 100 years after that, the British rock band Coldplay released their Music of the Spheres album, with each track representing a celestial body. Clearly, the impact of this spiritual, philosophical, mathematical, metaphysical, scientific, and astronomical concept has resonated throughout the millennia. I, for one, am standing by to enjoy the ongoing evolution and wonderment of this sonic journey.