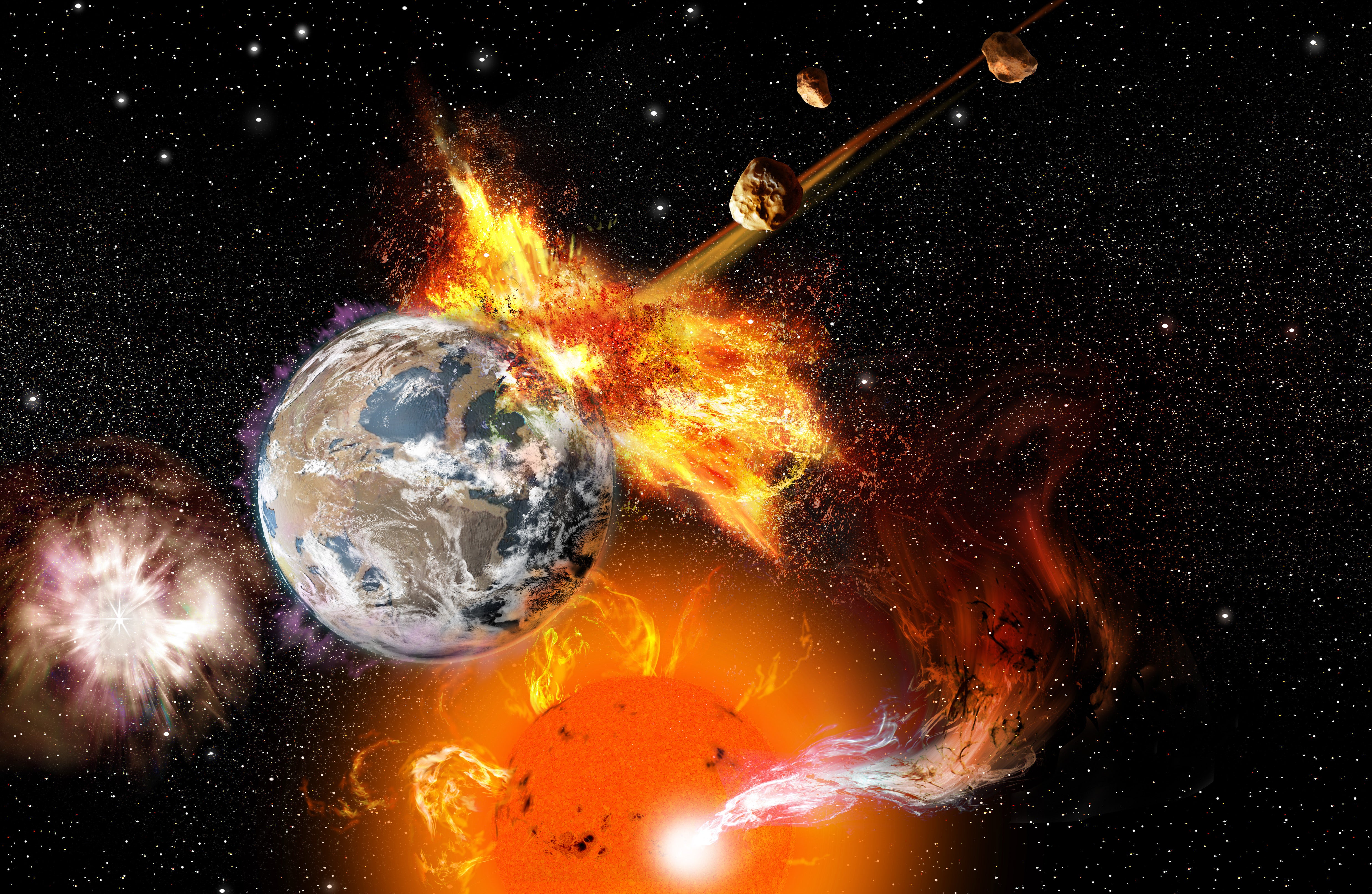
The universe is a terrifying place, filled with existential threats. Earth may seem quite solid beneath our feet, but the continued existence of the thin layers of rock, water, and air that sustain us is in no way guaranteed. Errant asteroids, soaring superflares, and exploding supernovae are just a few of the calamities that might befall our fragile world.
In the short term, we may be able to manage or mitigate some of these threats. Asteroids can be diverted and power grids hardened. But other apocalypses are inevitable as the solar system ages: a runaway Moon, Earth’s collapsing magnetosphere, the Sun’s flagging heart. Each one represents a countdown to a different apocalypse, with some more imminent than others.
Moving mountains
The most obvious threat is the one that has been featured in countless sci-fi stories and films: asteroids. Most famously, 66 million years ago, a miles-wide asteroid slammed into the ocean near the Yucatán Peninsula and plunged the planet into chaos. Wildfires that consumed continents and bone-chilling nuclear winters ended the 180-million-year reign of giant reptiles in the geological blink of an eye.
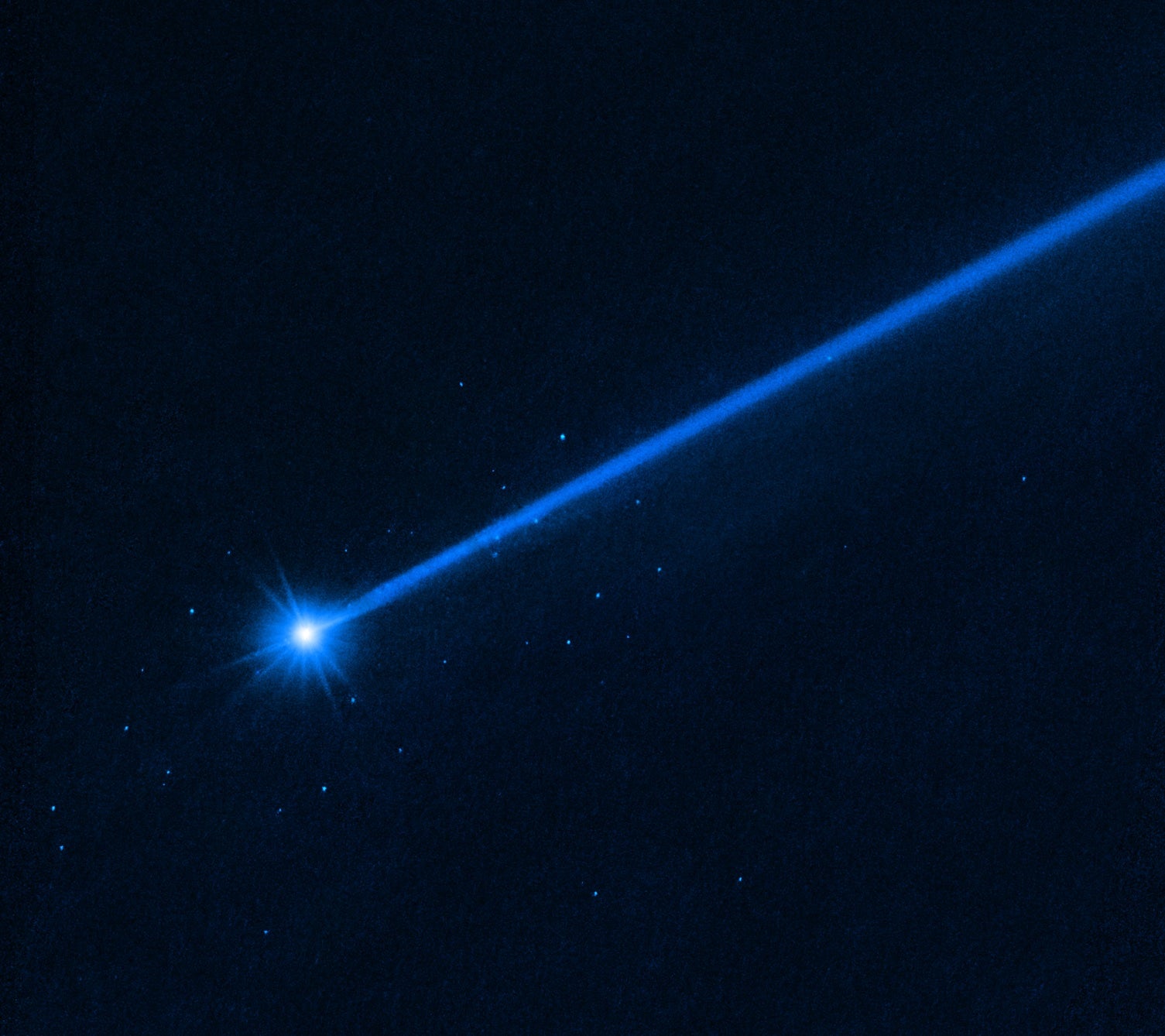
Until recently, we were no better prepared to ward off these collisions than the dinosaurs. But that changed in September 2022 when NASA’s Double Asteroid Redirection Test (DART) proved that humans could, in principle, deflect asteroids from catastrophic collisions with Earth. Weighing just over half a ton, the DART spacecraft smashed into an asteroid called Dimorphos, the junior partner of a binary system, at nearly 14,000 mph (22,500 km/h) — generating the energy equivalent of three tons of TNT.
The results of the experiment have been both enlightening and sobering.
“The impact of DART was just a tiny event in the life of this asteroid,” says David Jewitt, a professor of astronomy at the University of California, Los Angeles, who published a study about DART’s aftermath in July 2023. “If you wanted to deflect a bigger asteroid — for example, something 10 times larger — then you’d need 1,000 DARTs to get the same minuscule deflection. This deflection business is very, very tough.”
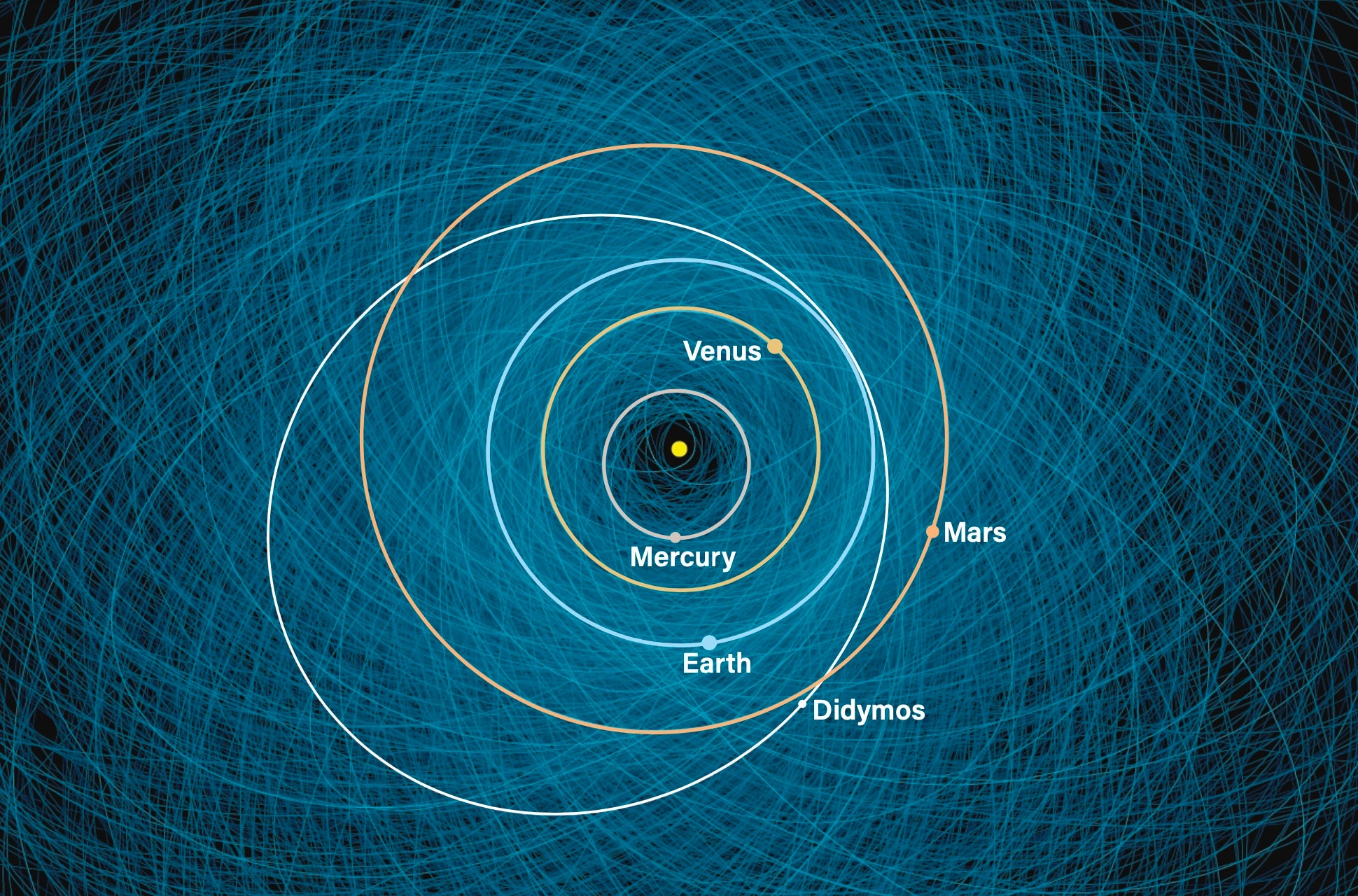
One of the reasons NASA chose Dimorphos is that it is locked in a binary system with an asteroid called Didymos — which, at half a mile (0.8 kilometer) wide, is five times Dimorphos’ size. This made any change generated by the impact easier to track. “The idea is that you hit a binary that has an initial orbit period of about 12 hours, and change that by a few minutes every orbit,” says Jewitt. “Even though the deflection is incredibly small, after 100 orbits it becomes a very measurable quantity. That’s what gave us success.”
As it turned out, the impact shortened the system’s 12-hour orbital tango by 32 minutes. Compared to the tens of miles per second at which the 5.5-million-ton Dimorphos whips around the Sun, this translates to a tiny fraction of its momentum — tenths of an inch per second. Jewitt says that any useful deflection of a larger asteroid would require a far greater shove or need to occur decades ahead of an impending terrestrial collision to have a cumulative effect.
A larger impact, however, means more debris — and more headaches. As Hercules saw, decapitating the Hydra only multiplies its deadly heads. Months after the DART collision, Jewitt and colleagues studying Hubble images discovered a swarm of previously undetected boulders, some as large as houses, drifting away in all directions at an average speed of 0.6 mph (1 km/h).
“It’s possible they were blasted out in the same way as all the smaller debris,” says Jewitt, “but it’s also possible that, because of the very low gravity, the boulders that were preexisting on the surface were shaken off.”
The difference matters. If we hope to intercept incoming asteroids in nondestructive ways, we need to know how to execute a gentle nudge. Jewitt believes that the boulders are blast collateral, but he won’t know until July, when the binary system returns to Hubble’s view. Comparing the previously charted trajectories of the boulders with new imagery, he and his colleagues hope to reconstruct how they were cast into space.
After that, the boulders will not be visible from Earth for 15 years, until Dimorphos and Didymos’ orbit brings them closer to Earth. However, they will be visited in the interim by the European Space Agency’s (ESA) Hera spacecraft in late 2026.
Even assuming we could deflect an incoming object, the challenge remains to find potentially hazardous asteroids with the months to years of advance notice needed to mount a mission. NASA’s Center for Near-Earth Object Studies currently lists only a few space rocks of immediate concern, but new ones show up frequently with little warning. With barely a day of advance notice, asteroids whipped past Earth in 2012, 2019, and 2021, ranging in size from a football field to several city blocks. The 11,000-ton Chelyabinsk meteor that exploded over the Russian Urals in 2013, damaging thousands of buildings and injuring over 1,000 people, was not on anybody’s radar.
This lack of awareness should begin to change with the opening of the Vera C. Rubin Observatory in Chile in 2025 and the launch of NASA’s Nancy Grace Roman Space Telescope in 2026. Rubin will be able to survey the entire southern sky every three days, generating 20 terabytes of data each night and issuing on average 10 million alerts regarding any detected changes — all processed and shared worldwide in less than 60 seconds. Roman will pack the same crisp sub-arcsecond resolution as Hubble, but with 100 times the field of view, generating a separate mountain of data on changing and moving objects in the sky.
When the two join forces, both hemispheres of the sky will come under intense scrutiny for everything from asteroids to supernovae.
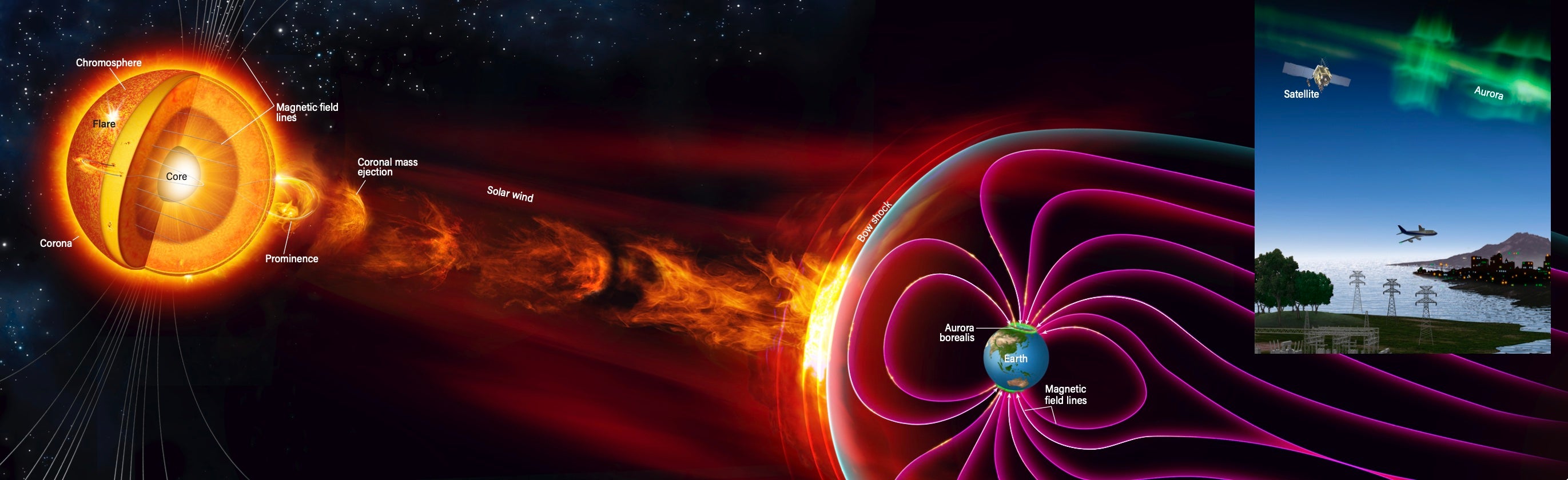
Bracing for a flare-up
Even as Rubin and Roman scour the skies, another doomsday clock continues ticking: solar superflares. Solar flares are commonplace and generally benign. They are outbursts of light and radiation launched from regions of the Sun with intense magnetic fields, whose endpoints are marked on the solar surface by the cooler dark patches known as sunspots.
Above sunspots, the Sun’s magnetic field can stretch out for tens of thousands of miles, carrying tendrils of superheated, magnetically bound plasma. The magnetic field lines store energy like stretched and twisted rubber bands — and when they snap, they can release huge quantities of plasma, called coronal mass ejections (CMEs). If a CME happens to be aimed at Earth, a geomagnetic storm will hit us days later. Thanks to the protective magnetosphere generated by Earth’s molten iron core, most CMEs are harmlessly deflected.
But once in a great while, a flare hundreds or thousands of times more powerful than normal — a superflare — belches a wallop that penetrates Earth’s magnetosphere.
Analysis of radiocarbon spikes preserved in the rings of ancient trees shows that in the past 10,000 years, at least six such solar storms have showered Earth. These are named Miyake events after physicist Fusa Miyake, who in 2012 reported the first such event, detected in Japanese cedar tree rings from 774 c.e. Subsequent studies uncovered another spike in 993 c.e., and then four more in the years 663, 5259, 5410, and 7176 b.c.e.
There is still much debate surrounding the exact nature of Miyake events. A paper published in October 2022 suggests that some Miyake events may be series of consecutive solar storms within a given year. But the leading explanation for these occurrences are superflares from our Sun.
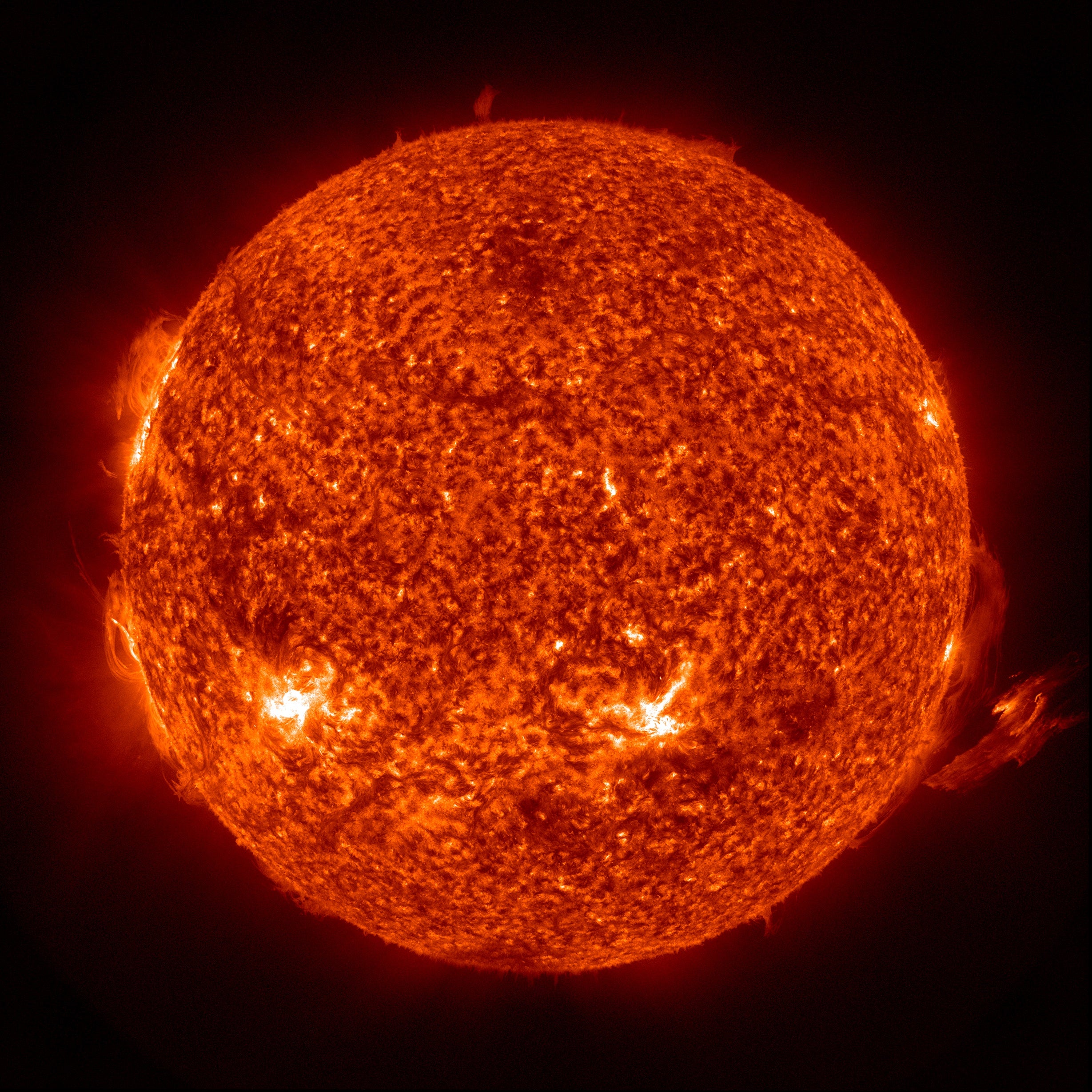
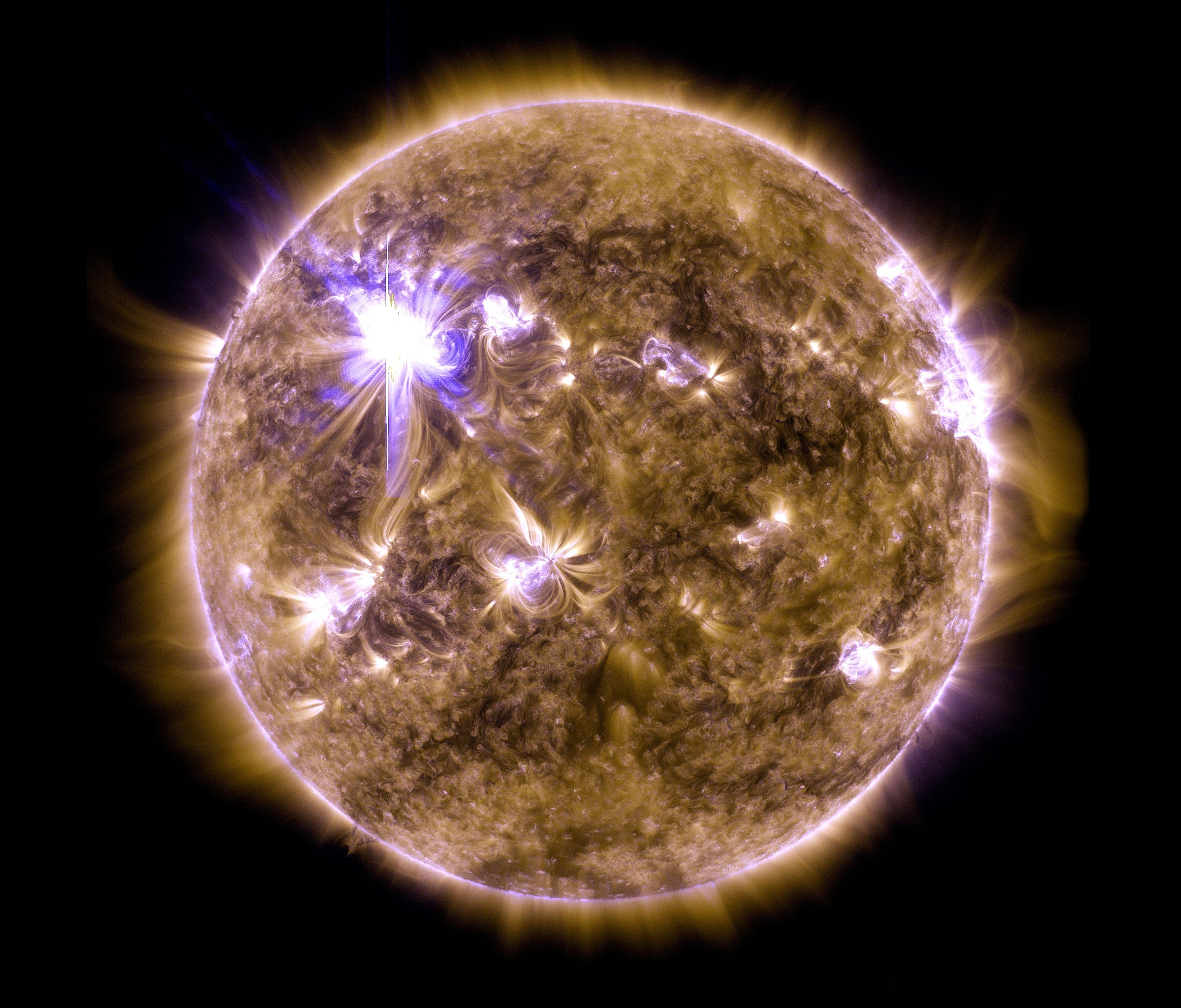
To our prehistoric ancestors, these events likely went unnoticed, aside from spectacular auroral light shows at unusually low latitudes. But in modern times, CMEs from superflares pose a serious threat. They could hobble satellites, crash GPS systems, and disrupt global communications. Electrical grids could overload and take months to be rebuilt. Cellphones, laptops, and other electronic devices would survive, but many would be limited by the lack of functioning telecommunications.
Modern civilization already experienced a small dose of these consequences in 1989, when a sizeable CME glanced across Québec, frying high-voltage lines and causing widespread blackouts. The last superflare before that was the Carrington Event of 1859; the ensuing geomagnetic storm sent currents coursing through telegraph wires, setting fires in telegraph offices across the U.S.
To learn how to predict superflares, astronomers are looking not just at the Sun but also to other stars. Young, hot, massive stars that burn blue and spin quickly can generate superflares at the blazing pace of one a day. Our Sun, a yellow dwarf, is much cooler and longer lived, so superflares are relatively rare.
But how rare? In 2019, a team led by Yuta Notsu of the University of Colorado Boulder used data from NASA’s Kepler space telescope and the ESA’s Gaia star-mapping satellite to determine that on Sun-like stars, Carrington-size flares occur every 500 to 600 years. High-end superflares — 100 times more powerful than Carrington — arise every 2,000 to 3,000 years.
By that measure, we aren’t yet overdue for another Carrington Event. But the Federal Energy Regulatory Commission is hedging its bet. In 2013, it issued Order 779 directing the North American Electric Reliability Corporation, a nonprofit power grid overseer, to develop plans for “blocking geomagnetically induced currents from entering the Bulk-Power System.”
Observing superflares in other star systems also gives a dramatic sense of the threats any other life-forms in the galaxy might face. In another sobering paper that Notsu co-authored in April 2023, he and colleagues described the largest superflare and prominence ever recorded in real time using ground- and space-based telescopes — albeit in a binary star system (V1355 Orionis) unlike our Sun.
“The observed velocity of that prominence far exceeded the escape velocity of its star,” says Notsu, “indicating that the eruption was capable of becoming a CME. Such eruptions are very important for evaluating the potential effects on planetary atmospheres.”
Notsu notes that the size and speed of the ejected material — trillions of tons traveling at 3 million mph (4.8 million km/h) — shows how large CMEs can get. The V1355 Orionis event was 10 times more powerful than anything the Sun is known to have ever produced, potentially enough to strip any nearby planets of their atmospheres.
Stardust to stardust
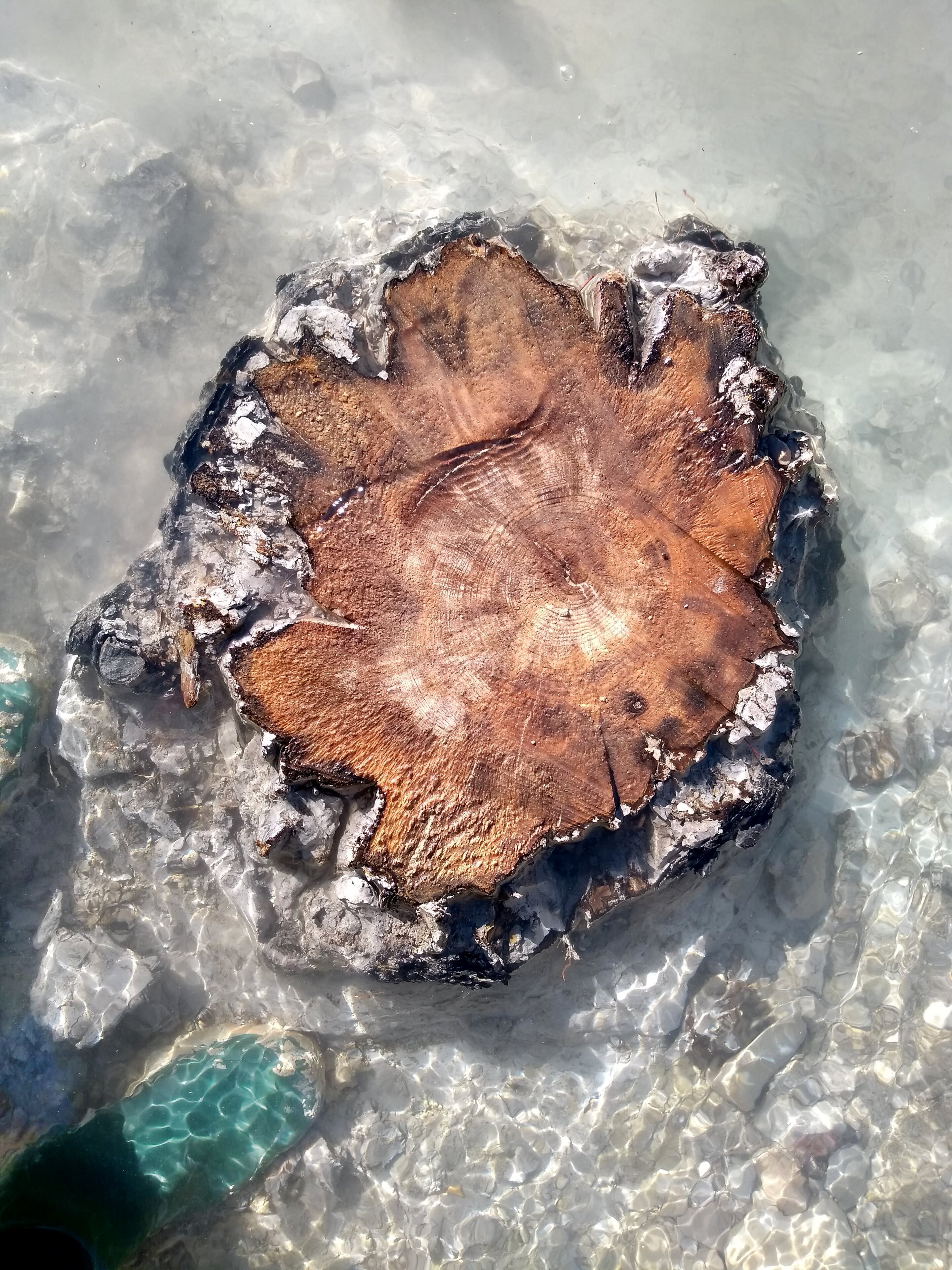
Perhaps nothing symbolizes the cosmic cycle of life and death like a supernova. These events are either the explosive final act of a massive star or a white dwarf triggered by runaway nuclear fusion. Just like asteroids and superflares, there is ample evidence that supernovae have bombarded Earth and even shaped life throughout our planet’s history.
“We are the children of supernovae,” says Brian Fields, an astronomer at the University of Illinois Urbana-Champaign. “Life would not be possible without them. The iron in your blood, the oxygen you’re breathing, the silicon in your computer, all are from supernovae that blew up long before the solar system was formed.”
While supernovae provide the building blocks of life, they can also destroy it. Being too close can mean instant incineration or lethal irradiation. Although the gamma rays supernovae produce cannot penetrate the upper reaches of our atmosphere, they can destroy our protective ozone layer through a chain of chemical reactions.
There are several types of supernovae, but it is the death throes of high-mass stars — weighing at least eight times our Sun — that spark the colossal core-collapse or type II supernovae. These supernovae produce nearly all atomic elements besides hydrogen and helium. Within our Milky Way Galaxy, two or three supernovae occur every century.
When shrouded in gas, type II supernovae become particularly potent. Months or years after the initial burst of gamma rays, superheated shock waves reverberate within the gas bubble and generate copious amounts of X-rays. Millennia later, as the blast barrels through space, it interacts with dust and radiation, producing high-energy particles called cosmic rays.
To understand the reach and frequency of these multistage killer events, scientists need more than Miyake dating. Tree-ring records go back only 15,000 years and carbon-14 dating isn’t reliable after 60,000 years (too much of the sample has decayed away), so uncovering traces of nearby supernovae in the geological record requires different techniques.
In 2015, a team of scientists perfected the use of an isotope called iron-60, a byproduct of supernovae, as a powerful chronometer with a half-life of 2.6 million years, providing reliable dates up to 10 million years in the past.
“It’s basically like tree rings,” says Fields. “We know there were recent nearby supernovae explosions because there is a wealth of iron-60 in two specific layers on the ocean floor as well as on the Moon and in Antarctic snow. Something brought it here 3 million years ago, and another pulse 7 million years ago.”
While iron-60 does an excellent job of detecting past supernovae, it can also help determine how far away they were. “The farther away you stand, the less iron-60 you intercept,” says Fields. “By seeing how much is there, we can work out the distance.”
Until a recent paper that Fields co-authored with principal investigator Ian Brunton, scientists deemed 10 parsecs — about 33 light-years — to be a safe enough distance for surviving supernovae. Their new research significantly expands the kill zone for gaseous type II supernovae to about 160 light-years, multiplying the affected volume of space about 125 times over.
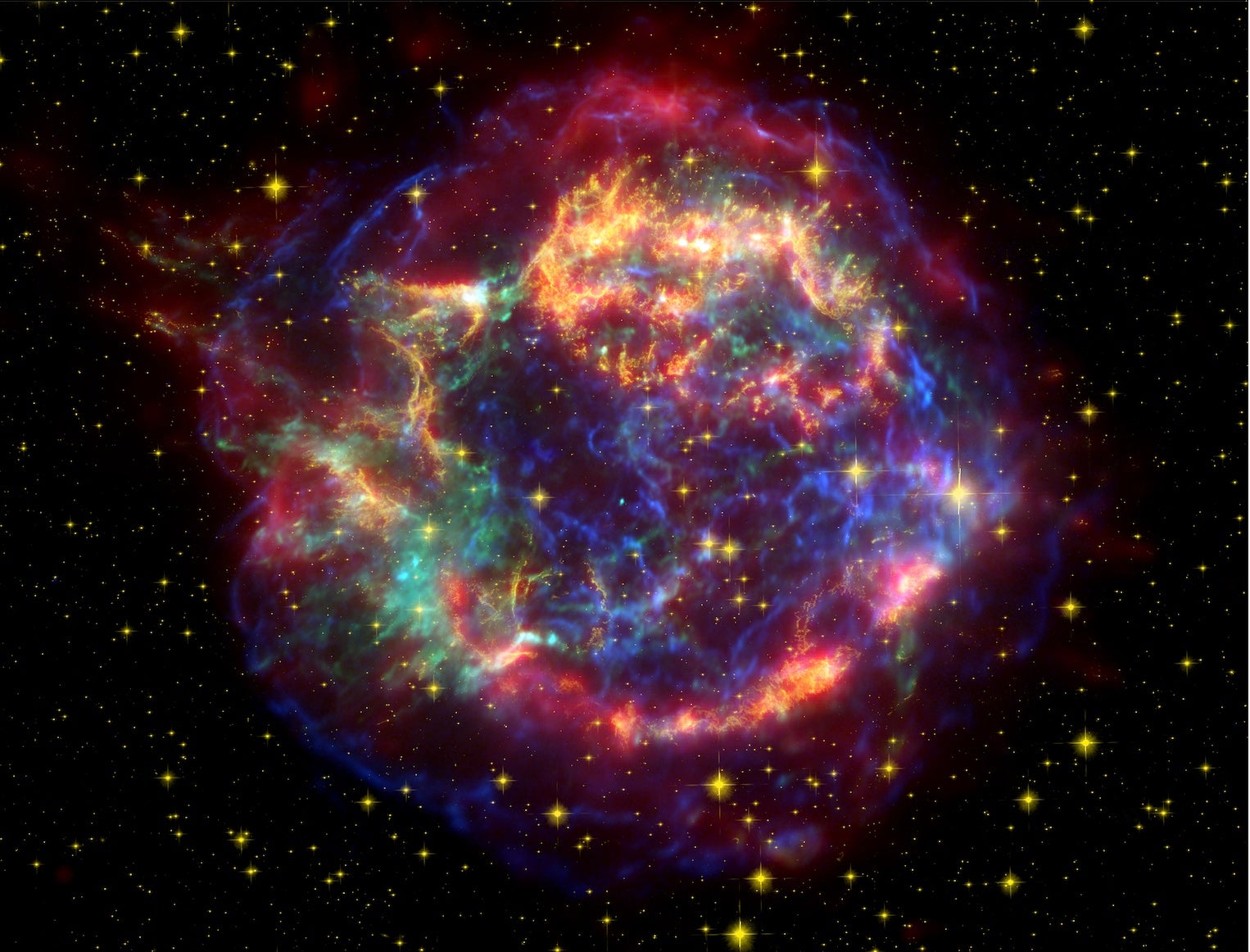
“That’s why we wrote the paper,” says Fields. “We realized that this kind of supernova can be dangerous to a much larger region than ordinary supernovae.”
Fields notes that the supernova 3 million years ago coincided with a notable megafauna die-off in the Pliocene.
According to one theory, muons may be the reason. These subatomic particles, about 200 times heavier than electrons, are produced by gamma rays. Large megafauna even half a mile (0.8 km) underwater would have been especially subject to their lethal effects. Based on iron-60 traces in seafloor sediments, astrobiologists have linked the die-off of whale-sized megalodon sharks with at least one supernova 2.6 million years ago in the Local Bubble, some 160 light-years away, at that very time.
The good news is that because supernova candidates are supermassive stars, they are also hard to miss, pumping out 100,000 times more light than the Sun. When they’re nearby, we know it, and even those shrouded in gas are brilliant in infrared wavelengths.
When this next occurs, it won’t likely be from any star we know by name today. Spica in Virgo is the nearest supermassive star likely to go supernova, and it lies some 250 light-years away — well outside the supernova danger zone.
But as our star system orbits the center of the Milky Way, it passes through our galaxy’s spiral arms every 100 million years or so. By some estimates, each passage is likely to bring Earth within 33 light-years of a supernova, leaving it potentially exposed to its devastating effects.
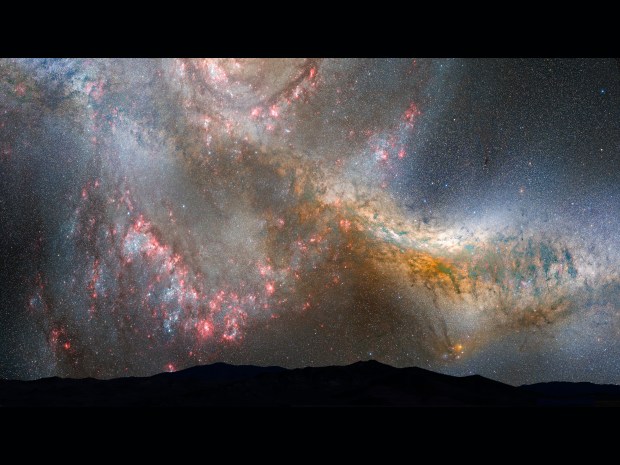
March of time
Beyond Earth’s most pressing cosmic countdowns, the faltering dynamics of an aging solar system await. They are far in the future — several times as long as life itself has populated our planet — but they are fascinating to contemplate.
The first to go could be our planet’s magnetic core. A January 2022 study of thermal conductivity at Earth’s core-mantle boundary found that a mineral called bridgmanite is transferring heat out of the core 50 percent faster than previously thought. Once our core cools enough, its magnetic properties will fail. Without the magnetosphere to protect us, solar storms will gradually strip away our atmosphere.
While the atmosphere decays, the Moon’s tidal pull will continue sapping Earth’s momentum. The law of conservation of angular momentum dictates that Earth’s deceleration will accelerate the Moon, launching it farther outward. Total eclipses will become mere transits as the Moon tidally locks over a single point on one side of the Earth. This dance will then reverse as gravity draws Earth and Moon together again, ultimately ripping our satellite asunder and throwing our orbit and seasons into disarray.
Meanwhile, the evolving Sun will play havoc with Earth and its temperature regulation. As stars like the Sun mature, they burn brighter — by about 10 percent every billion years. This will push the solar system’s habitable zone outward, beyond Earth. A billion years from now, Earth will be too hot to maintain liquid water on its surface. The oceans will evaporate, thickening the atmosphere into an oppressive greenhouse and leaving behind a barren, scorched landscape.
Prospects for any surviving life are grim. Eventually, the Sun will lose its nuclear gusto when the hydrogen supply in its core runs out. As its now-helium core contracts, a shell of hydrogen around the core will temporarily ignite. The Sun will swell into a red giant and its fiery outer layers will consume Earth’s orbit (but fall short of Mars). For a few million years, the moons of Jupiter and Saturn may sit in the habitable zone.
Ultimately, the outward pressure of the Sun’s radiation will weaken and its own gravity will trigger its demise. Five billion years from now, the Sun’s core will collapse into a small, dense white dwarf, even as its outer layers are expelled into a planetary nebula. At about the same time, the Milky Way and Andromeda galaxies will collide in a spectacular maelstrom of light and energy. Eventually the merged galaxies will settle down as an elliptical galaxy, a homogenous ball of aging suns with little dust, gas, or new star production.
Finally comes the fate of the universe itself. Scenarios range from a Big Crunch — the reversal of the Big Bang toward a new singularity — to a Big Freeze or Big Rip, an infinite expansion driven by dark energy that flings stars and galaxies so far apart that the night sky becomes a blank slate. A June 2023 paper suggested an alternate finale in which gravity and the theoretical force known as Hawking radiation cause all matter in the universe to simply evaporate, starting with black holes.
Contemplating these catastrophes and the ticking clocks that mark their inexorable approach can be a bit of a downer, no doubt. But perhaps we should instead count ourselves lucky, and savor this moment in the universe’s chronology — a brief window between clock resets — that has allowed life on our planet to flourish.