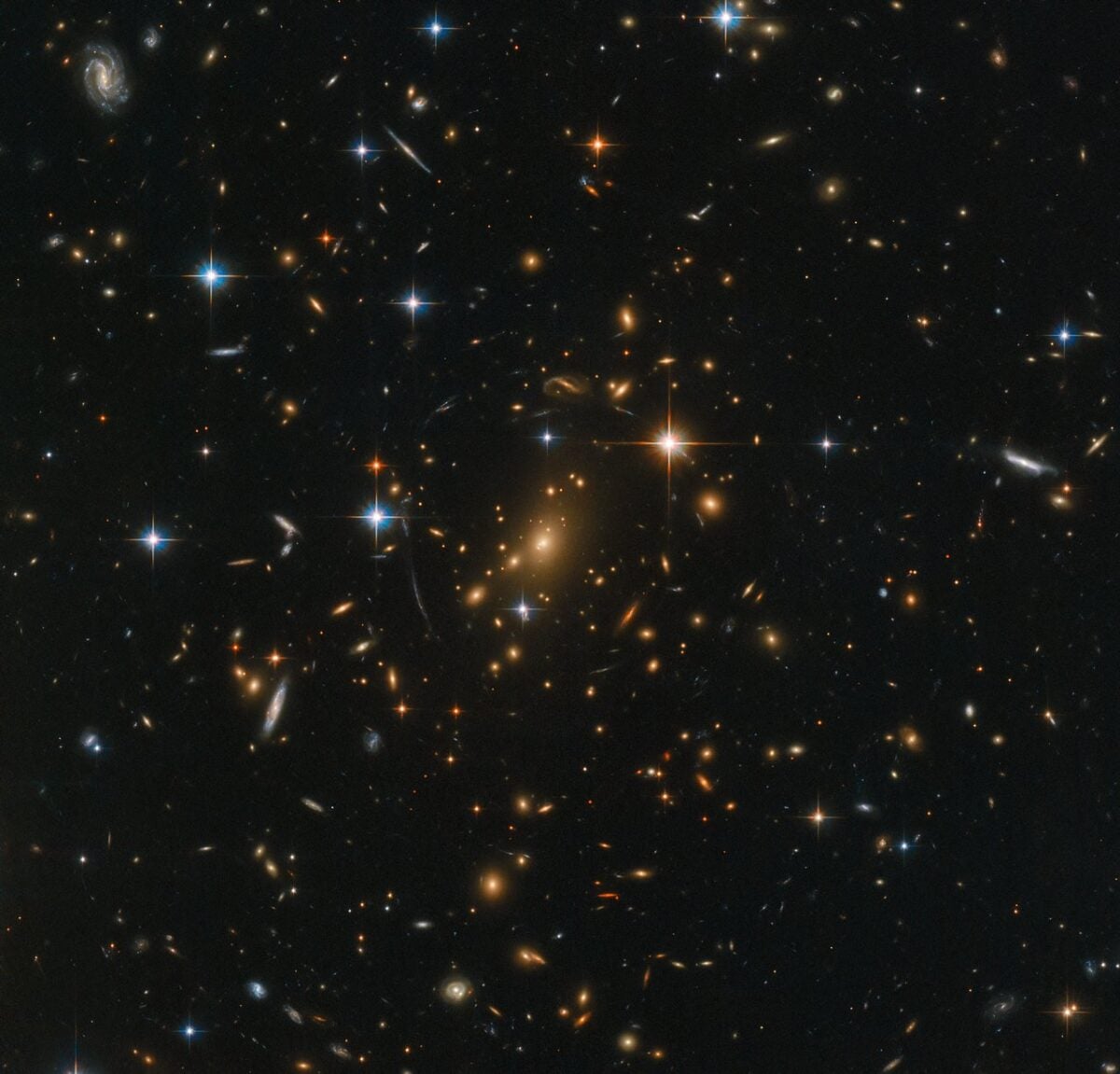
Our universe is shrouded in mystery, with about 70 percent of it consisting of dark energy. The exact nature of dark energy remains a puzzle that, once solved, could unlock profound insights into the formation of our solar system, the evolution of the Milky Way Galaxy, the origins of life, and even the fate of our universe.
Dark energy can be described as the effect of a negative pressure pushing space outward. We will get more technical, I promise, but let’s start with an analogy to understand this fundamental concept. Imagine you have a balloon representing the entire universe, and you place stickers all over its surface to represent galaxies or clusters of galaxies. Imagine the first pump of air into the balloon — this equates to the Big Bang and initiates the beginning of time as we know it. As the balloon expands and time passes, the distance between any two stickers (or galaxies) increases. This is similar to how galaxies are moving farther apart from each other, showing evidence for an expanding universe.
Not only is the universe expanding, but it is expanding at an accelerated rate, meaning it is getting bigger faster and faster over time. In our balloon analogy, imagine blowing air into the balloon rapidly, making it expand faster and faster. This additional pressure represents dark energy pushing the balloon (or our universe) to grow at an accelerated rate.
Dark energy and dark matter
Before diving deeper into the depths of dark energy, you might be asking what the difference between dark energy and dark matter is, or how they might be similar. Dark energy and dark matter, the enigmatic duo, make up about 96 percent of the universe (roughly 70 percent dark energy and 26 percent dark matter). Meanwhile, baryonic, or normal, matter, such as stars, plants, cats, you, and me, only accounts for about 4 percent of the universe’s contents.
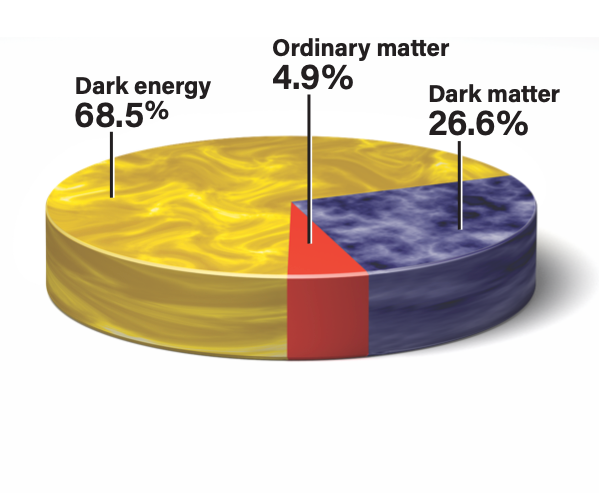
Related: Are the percentages of dark matter and dark energy stable?
While the terms are often confused, the effects of dark energy and dark matter are very different. Dark energy is a theoretical form of energy that counteracts gravitational attraction and drives or “pushes” the universe’s accelerated expansion. On the other hand, dark matter exerts a gravitational influence that can be detected through its effects, which “pull” together stars and galaxies, affecting their motions.
While their effects differ, they both earn their mysterious first name, “dark,” because we cannot directly observe them. Neither emits, absorbs, nor reflects light. Yet, we can observe how dark energy and dark matter interact with normal matter. This allows astronomers to achieve the seemingly impossible and study something we can’t see.
Detecting the unseen
Dark energy’s acceleration effects were first discovered by observing distant supernovae in the late 1990s. This was done with Type Ia supernovae, which act as “standard candles,” meaning they have a predictable luminosity. So, the fainter one of these supernovae appears, the farther away it is. This makes them valuable for measuring distances across vast cosmic epochs. By observing their brightness and redshift (a proxy for distance, which is affected by the expansion of the universe), scientists can trace the history of cosmic expansion and infer the influence of dark energy.
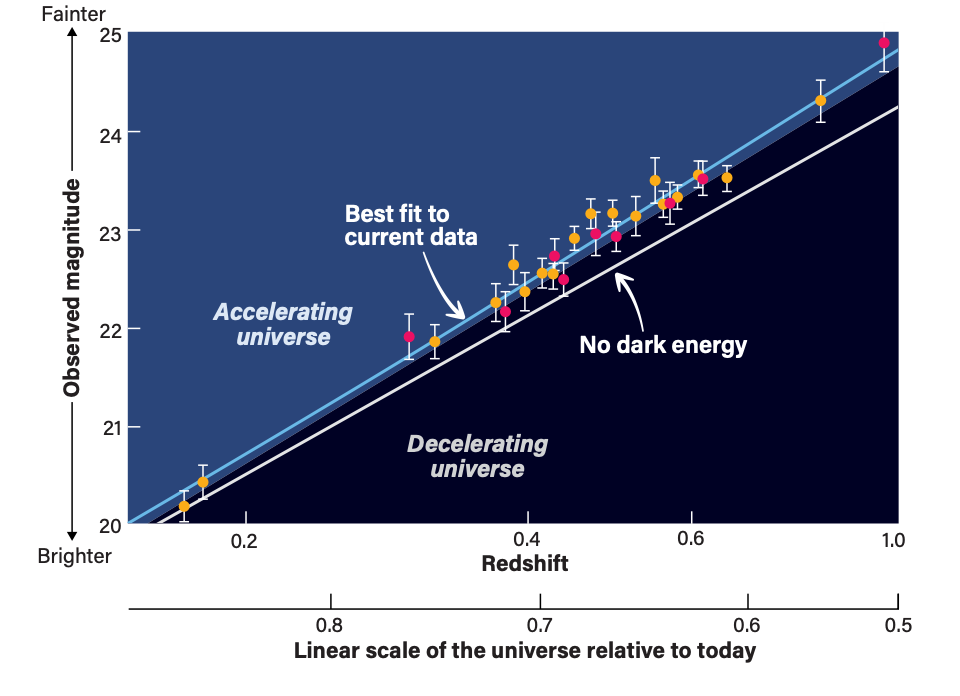
It can also be studied by examining baryon acoustic oscillations (BAOs). BAOs are regular fluctuations in the density of visible matter caused by pressure waves in the early universe. Essentially, they cause galaxies to cluster more strongly at specific scales, which then change during different epochs as the universe expands. So, BAOs also provide a “standard ruler” for measuring distances over cosmic scales. Large surveys do this by creating maps of the universe, allowing astronomers to study the scale of BAOs to trace the expansion history of the universe and constrain dark energy parameters.
Another innovative approach to understanding dark energy involves analyzing the bending of light (gravitational lensing) from distant galaxies and clusters. By analyzing the distortions in the shapes and positions of galaxies caused by gravitational lensing, scientists can probe the distribution of dark matter and further infer the properties of dark energy by observing how its influence affects structures throughout the universe.
Our future is in dark energy’s hands
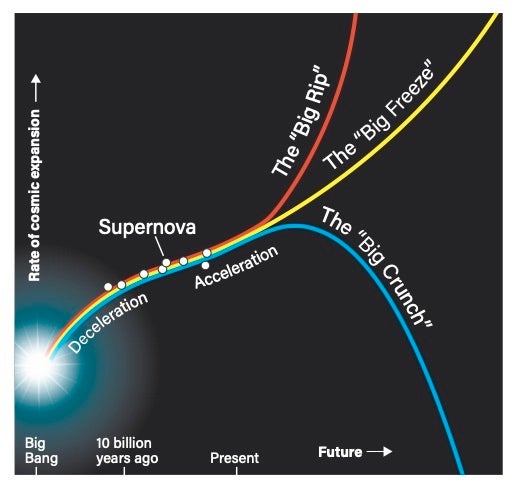
Ongoing and future surveys like the Dark Energy Survey (DES), the Legacy Survey of Space and Time (LSST), the Euclid mission, the Baryon Oscillation Spectroscopic Survey (BOSS), and the Hobby-Eberly Telescope Dark Energy Experiment (HETDEX) aim to expand our understanding of dark energy by observing larger volumes of the universe and refining cosmological measurements.
These multi-million-dollar, decade-long experiments might expose the answer to how dark energy has shaped our universe or how it may yet destroy the universe (or potentially reveal another!).
There are three theories about how dark energy could impact the fate of the universe. The first theory is heat death or the so-called Big Freeze. In this scenario, dark energy keeps pushing galaxies farther apart, causing the universe to become emptier and darker. Eventually, everything — including us if we’re still around — will reach a uniform temperature. To imagine a case like the Big Freeze, picture placing our balloon in the freezer. Eventually, with enough time, the balloon will reach the same temperature as the freezer, relinquishing all the properties we previously associated with how a balloon functions at room temperature. The same applies to our universe.
The second is a fascinating conjecture known as the Big Rip scenario. According to this hypothesis, the pervasive influence of dark energy will propel the universe’s expansion at an increasingly rapid pace. Ultimately, cosmologists theorize that this relentless acceleration will reach a point where it exerts a force so potent that it rends apart celestial entities. Equivalently, this is an example I’m sure all of us have experienced when blowing up a balloon. When you push too much air into the balloon too quickly, the balloon pops and is strewn around the room, leaving behind balloon fragments and stickers — or, in the cosmic case, galaxies and planets — that were blown apart.
The third scenario is called the Big Crunch, which is the opposite of expansion. It suggests that the effects of dark energy could change from causing the universe to expand to causing it to collapse. This would lead to everything collapsing in on itself and possibly resetting us back to the way things looked at the beginning of time. This would be like letting all the air out of our balloon and watching the chaotic universe fly around the room until it peacefully rests on the ground, waiting to be blown up again.