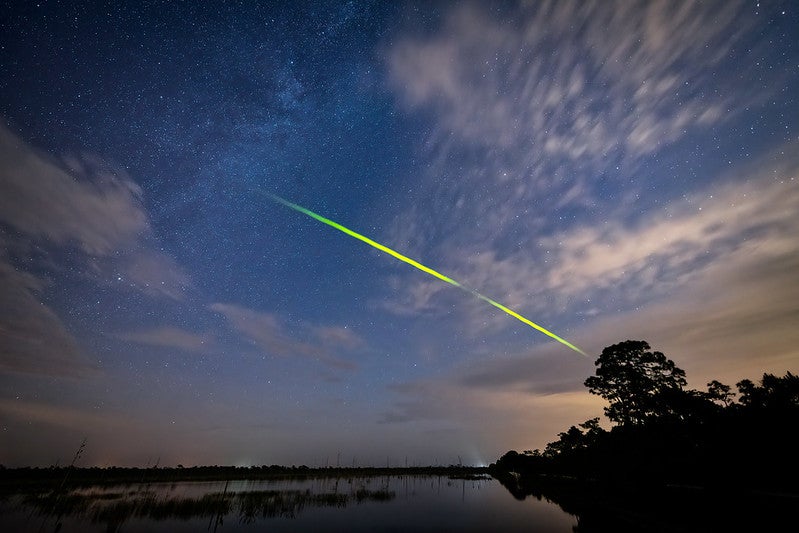
Much of what scientists know about the early solar system comes from meteorites – ancient rocks that travel through space and survive a fiery plunge through Earth’s atmosphere. Among meteorites, one type – called carbonaceous chondrites – stands out as the most primitive and provides a unique glimpse into the solar system’s infancy.
The carbonaceous chondrites are rich in water, carbon and organic compounds. They’re “hydrated,” which means they contain water bound within minerals in the rock. The components of the water are locked into crystal structures. Many researchers believe these ancient rocks played a crucial role in delivering water to early Earth.
Before hitting the Earth, rocks traveling through space are generally referred to as asteroids, meteoroids or comets, depending on their size and composition. If a piece of one of these objects makes it all the way to Earth, it becomes a “meteorite.”
From observing asteroids with telescopes, scientists know that most asteroids have water-rich, carbonaceous compositions. Models predict that most meteorites – over half – should also be carbonaceous. But less than 4% of all the meteorites found on Earth are carbonaceous. So why is there such a mismatch?
In a study published in the journal Nature Astronomy on April 14, 2025, my planetary scientist colleagues and I tried to answer an age-old question: Where are all the carbonaceous chondrites?
Sample-return missions
Scientists’ desire to study these ancient rocks has driven recent sample-return space missions. NASA’s OSIRIS‑REx and JAXA’s Hayabusa2 missions have transformed what researchers know about primitive, carbon‑rich asteroids.
Meteorites found sitting on the ground are exposed to rain, snow and plants, which can significantly change them and make analysis more difficult. So, the OSIRIS‑REx mission ventured to the asteroid Bennu to retrieve an unaltered sample. Retrieving this sample allowed scientists to examine the asteroid’s composition in detail.
Similarly, Hayabusa2’s journey to the asteroid Ryugu provided pristine samples of another, similarly water-rich asteroid.
Together these missions have let planetary scientists like me study pristine, fragile carbonaceous material from asteroids. These asteroids are a direct window into the building blocks of our solar system and the origins of life.
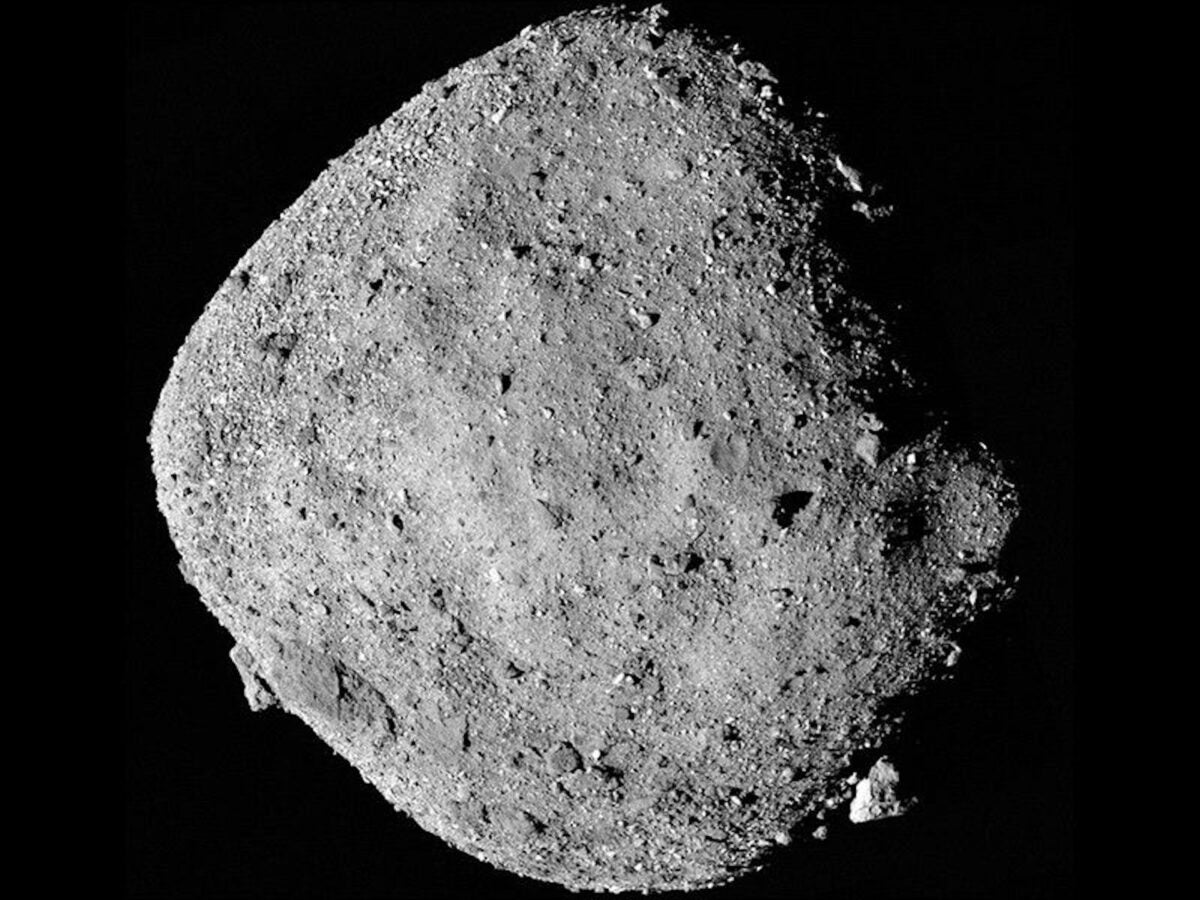
The carbonaceous chondrite puzzle
For a long time, scientists assumed that the Earth’s atmosphere filtered out carbonaceous debris.
When an object hits Earth’s atmosphere, it has to survive significant pressures and high temperatures. Carbonaceous chondrites tend to be weaker and more crumbly than other meteorites, so these objects just don’t stand as much of a chance.
Meteorites usually start their journey when two asteroids collide. These collisions create a bunch of centimeter- to meter-size rock fragments. These cosmic crumbs streak through the solar system and can, eventually, fall to Earth. When they’re smaller than a meter, scientists call them meteoroids.
Meteoroids are far too small for researchers to see with a telescope, unless they’re about to hit the Earth, and astronomers get lucky.
But there is another way scientists can study this population, and, in turn, understand why meteorites have such different compositions.
Meteor and fireball observation networks
Our research team used the Earth’s atmosphere as our detector.
Most of the meteoroids that reach Earth are tiny, sand-sized particles, but occasionally, bodies up to a couple of meters in diameter hit. Researchers estimate that about 5,000 metric tons of micrometeorites land on Earth annually. And, each year, between 4,000 and 10,000 large meteorites – golf ball-sized or larger – land on Earth. That’s more than 20 each day.
Today, digital cameras have rendered round-the-clock observations of the night sky both practical and affordable. Low-cost, high-sensitivity sensors and automated detection software allow researchers to monitor large sections of the night sky for bright flashes, which signal a meteoroid hitting the atmosphere.
Research teams can sift through these real-time observations using automated analysis techniques – or a very dedicated Ph.D. student – to find invaluable information.
Our team manages two global systems: FRIPON, a French-led network with stations in 15 countries; and the Global Fireball Observatory, a collaboration started by the team behind the Desert Fireball Network in Australia. Together with other open-access datasets, my colleagues and I used the trajectories of nearly 8,000 impacts observed by 19 observation networks spread across 39 countries.
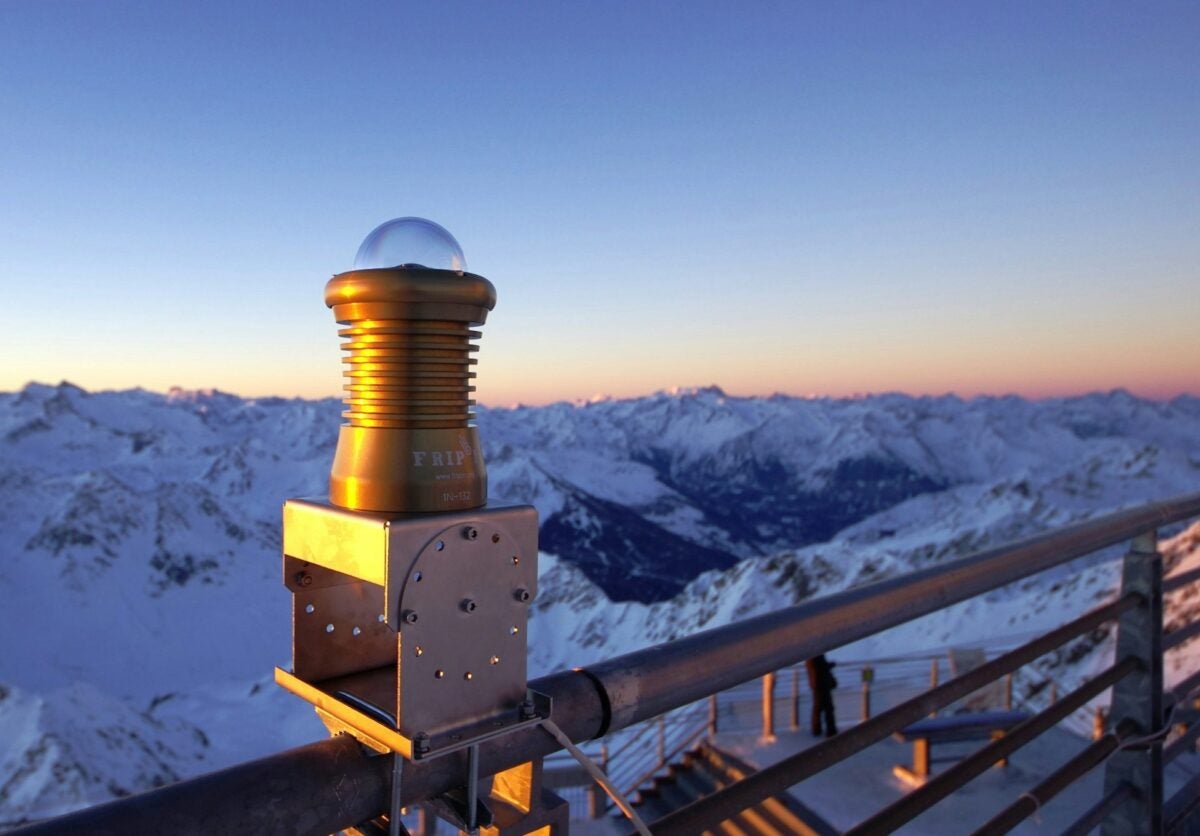
By comparing all meteoroid impacts recorded in Earth’s atmosphere with those that successfully reach the surface as meteorites, we can pinpoint which asteroids produce fragments that are strong enough to survive the journey. Or, conversely, we can also pinpoint which asteroids produce weak material that do not show up as often on Earth as meteorites.
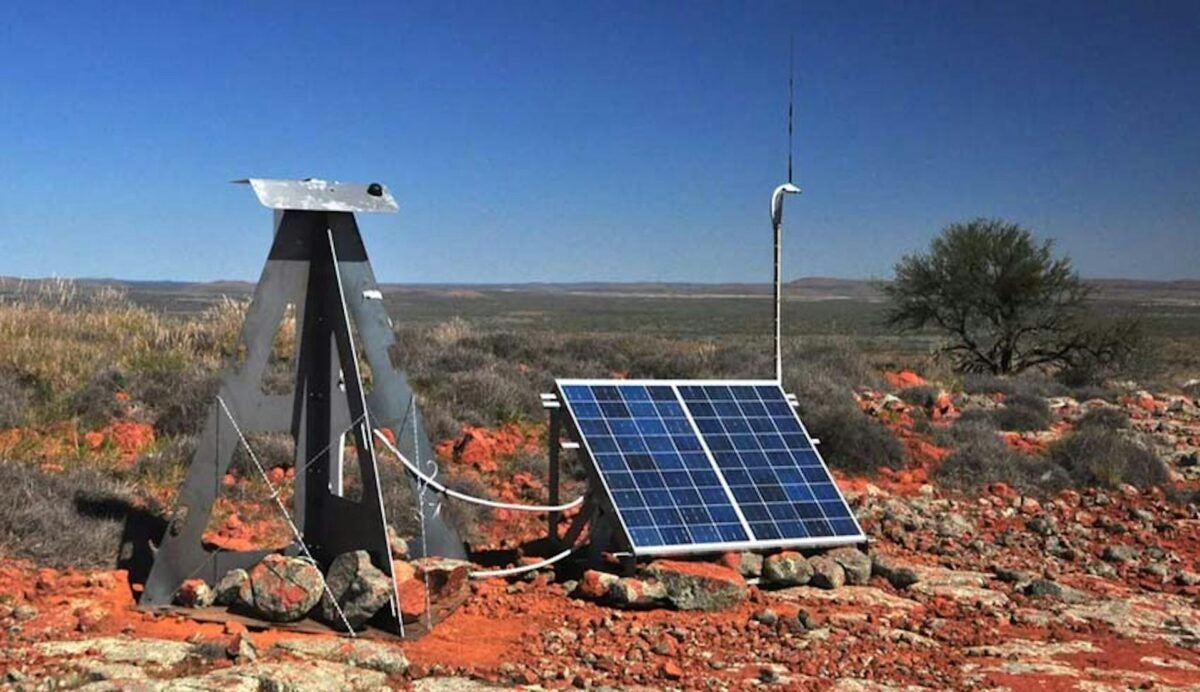
The Sun is baking the rocks too much
Surprisingly, we found that many asteroid pieces don’t even make it to Earth. Something starts removing the weak stuff while the fragment is still in space. The carbonaceous material, which isn’t very durable, likely gets broken down through heat stress when its orbit takes it close to the Sun.
As carbonaceous chondrites orbit close, and then away from the Sun, the temperature swings form cracks in their material. This process effectively fragments and removes weak, hydrated boulders from the population of objects near the Earth. Anything left over after this thermal cracking then has to survive the atmosphere.
Only 30%-50% of the remaining objects survive the atmospheric passage and become meteorites. The debris pieces whose orbits bring them closer to the Sun tend to be significantly more durable, making them far more likely to survive the difficult passage through Earth’s atmosphere. We call this a survival bias.
For decades, scientists have presumed that Earth’s atmosphere alone explains the scarcity of carbonaceous meteorites, but our work indicates that much of the removal occurs beforehand in space.
Going forward, new scientific advances can help confirm these findings and better identify meteoroid compositions. Scientists need to get better at using telescopes to detect objects right before they hit the Earth. More detailed modeling of how these objects break up in the atmosphere can also help researchers study them.
Lastly, future studies can come up with better methods to identify what these fireballs are made of using the colors of the meteors.

Patrick M. Shober is a Postdoctoral Fellow in Planetary Sciences at NASA.
Patrick M. Shober received funding from the European Union’s Horizon 2020 research and innovation program under the Marie Skłodowska-Curie grant agreement No. 945298. Patrick M. Shober currently receives funding from the NASA Postdoctoral Program.
This article was originally published on The Conversation. It is republished under a Creative Commons license.